This lecture can be viewed on YouTube site: Life Scinence Lectures for you
https://youtu.be/HXkCId2NHwE
1. Introduction
In this lecture, I explain the mechanisms of post transcriptional gene silencing by siRNA, miRNA, and piRNA.
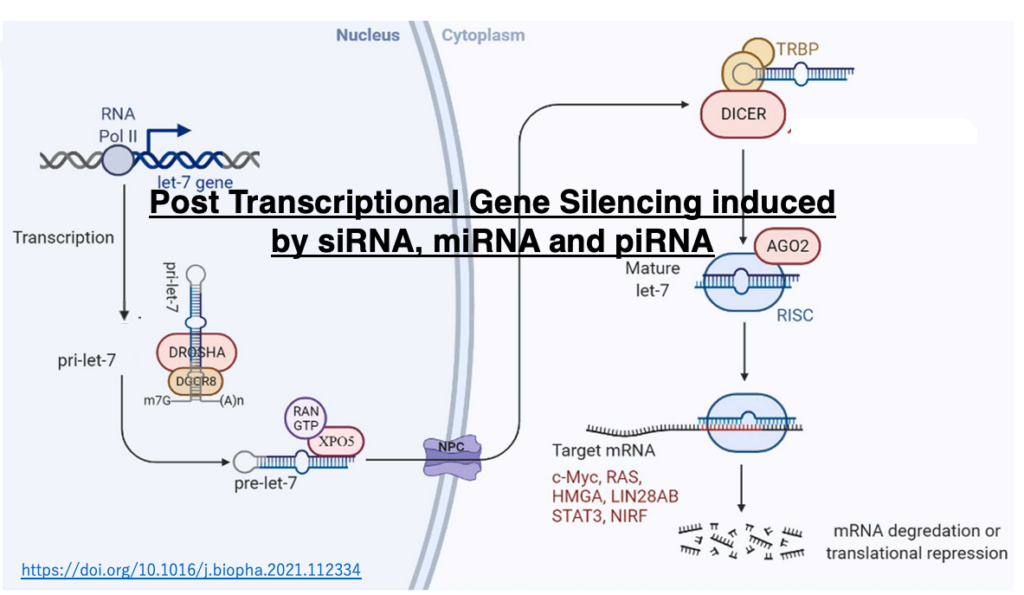
- The origin of RNA silencing, which exists only in eukaryotes
- RNA interference: post-transcriptional gene silencing involving siRNAs
- Generation of duplex siRNAs from long perfectly complementary RNA strands
- Cleavage of the passenger strand and its release from Ago
- Principles of guide strand selection
- Scanning of target mRNAs by the seed sequence of siRNAs
- Cleavage or translational inhibition of target mRNAs by RISC binding
- Mechanisms for producing secondary siRNAs
- Mechanism of post-transcriptional gene silencing by miRNAs
- Pathways of post-transcriptional gene silencing by miRNAs
- Comparison of post-transcriptional translational repression by siRNAs and miRNAs
- RNA silencing suppressors in plant viruses
- Mechanism of post-transcriptional gene silencing by piRNAs
- Comparison of piRNAs and miRNAs
- Pathway for cleavage of piRNA cluster transcripts and generation of activated Piwi-piRISC
- Pathway for production of mature piRNAs from transcripts of dual-strand clusters
Key words:RNA interference, siRNA, miRNA, piRNA, RNA silencing, Argonaute, RNA virus, RISC, TRBP, Guide strand, Passenger strand, secondary siRNA, germ cell, transposon, uni-strand piRNA cluste
2. Two pathways of RNA silencing
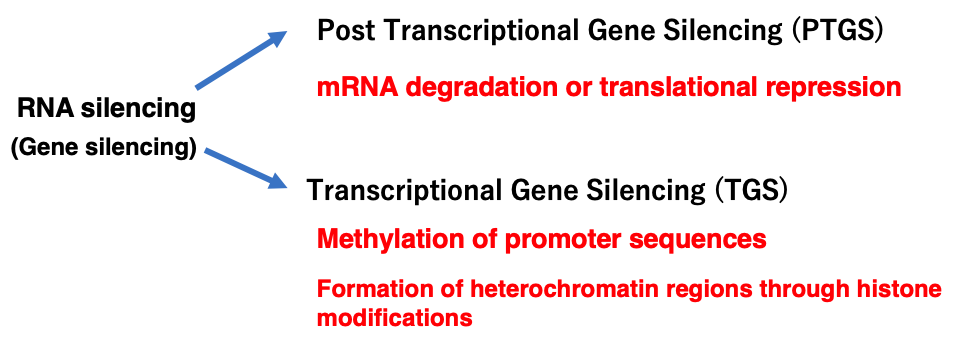
Non-coding small RNAs such as siRNA, miRNA, and piRNA are known to not only interfere with protein synthesis from mRNA through Post Transcriptional Gene Silencing, but also to induce transcriptional repression through the formation of heterochromatin regions via methylation of promoter sequences and histone modifications.
RNA silencing thus has two pathways, while transcriptional repression by small RNAs is not included in this lecture.
3. Origin of RNA silencing
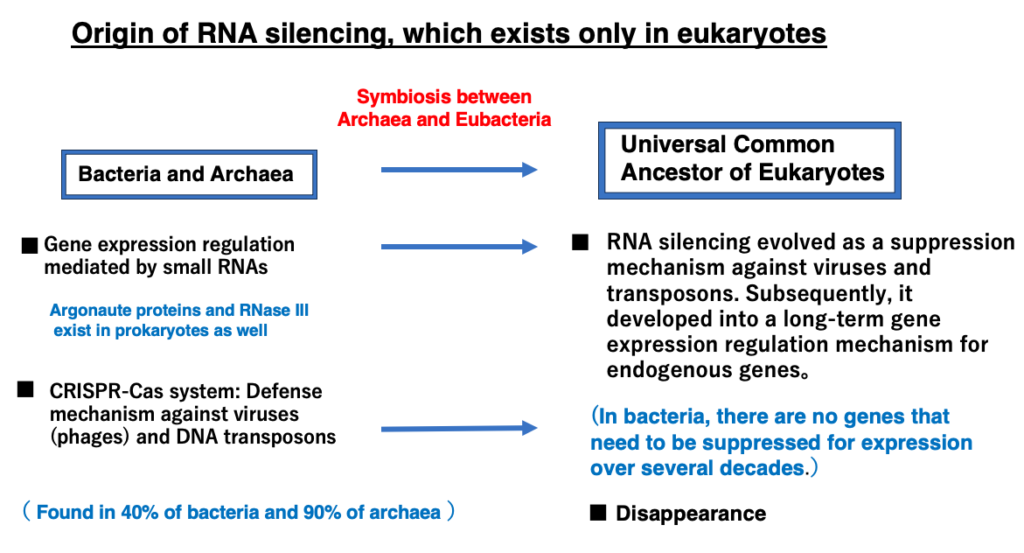
The origin of RNA silencing
RNA silencing exists only in eukaryotes, which does not exist in eubacteria or archaea. Since eukaryotic cells are hybrid cells that arose from the symbiosis of archaea and eubacteria, many mechanisms that are said to exist only in eukaryotes are often actually mechanisms that existed in bacteria, and have evolved and developed in eukaryotic cells.
4. Spread of RNA silencing in eukaryotes
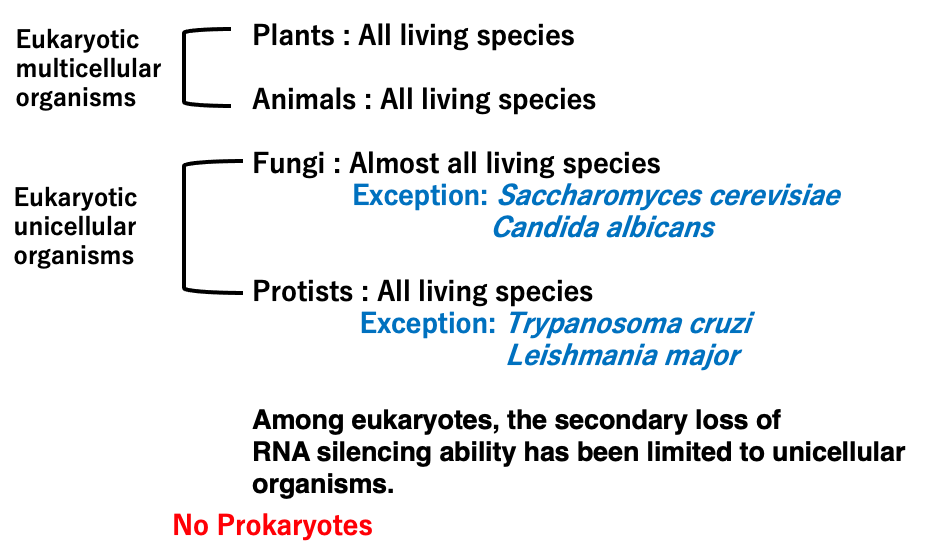
RNA silencing is observed in all multicellular eukaryotes including both plants and animals. It is also present in nearly all species of eukaryotic unicellular organisms such as fungi and protozoans. However, some of these unicellular eukaryotes are thought to have secondarily lost this mechanism.
For example, the budding yeast Saccharomyces cerevisiae lacks the RNA silencing response.
5. Long double-stranded RNA that serve as precursors to siRNA
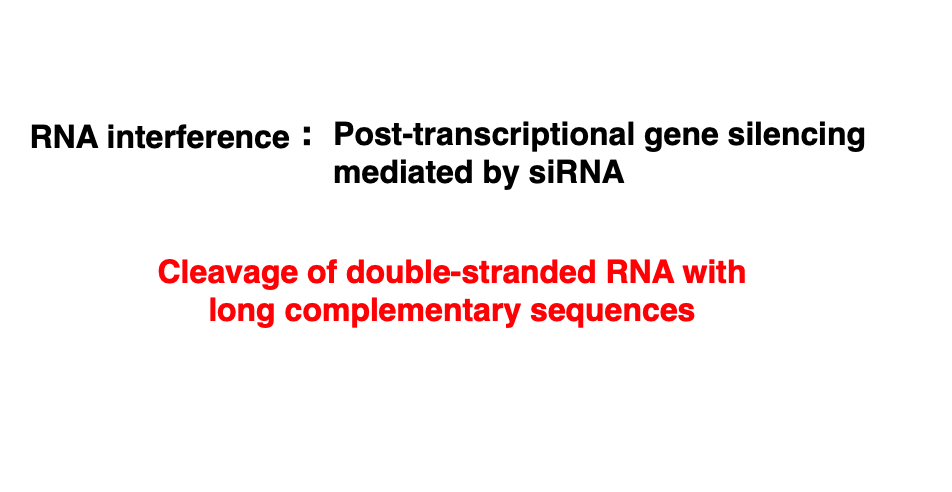
We begin the explanation of post-transcriptional gene silencing by short interfering RNA (siRNA) in RNA silencing. The precursor of siRNA is a long double-stranded RNA with complementary sequences. This is cleaved to produce siRNA.
6. RNA viruses that replicate through RdRp form long double-stranded RNA
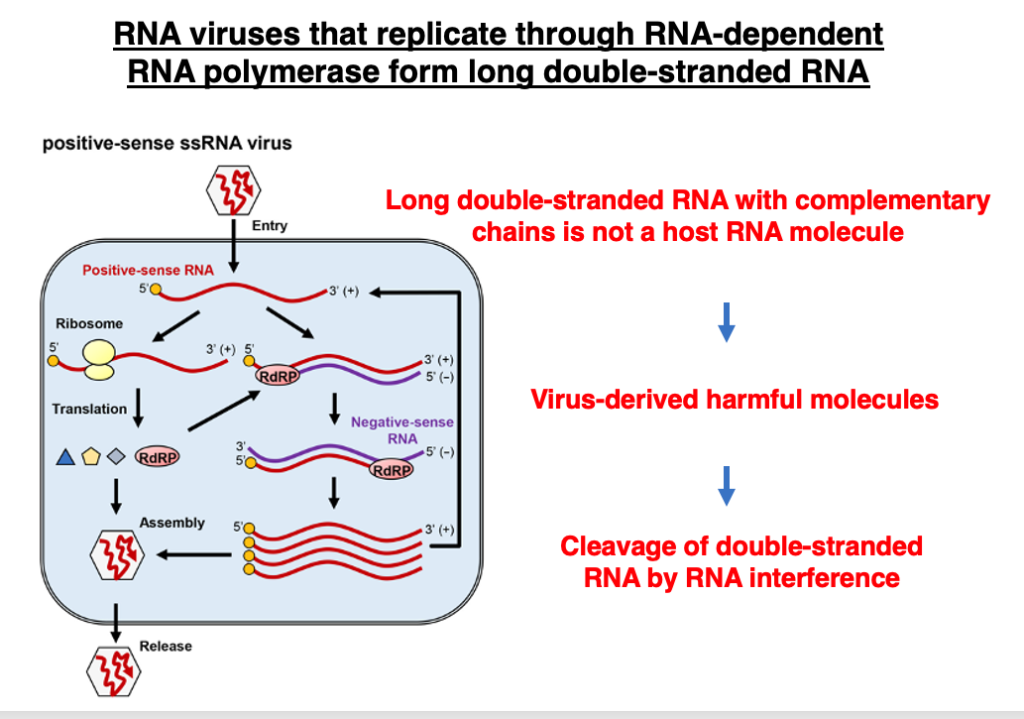
Long, perfectly complementary double-stranded RNAs derived from RNA viruses are targets for RNA interference. There are two types of RNA viruses: those that replicate by converting to DNA molecules via reverse transcriptase, and those that replicate using RNA-dependent RNA polymerase (RdRp).
Long, perfectly complementary RNA molecules produced by RNA-dependent RNA polymerase do not exist among transcripts of eukaryotic genes. Therefore, destroying such RNA molecules appears to be a beneficial mechanism for the host to eliminate replicating viral molecules.
7. Simplified RNA interference (RNAi) reaction
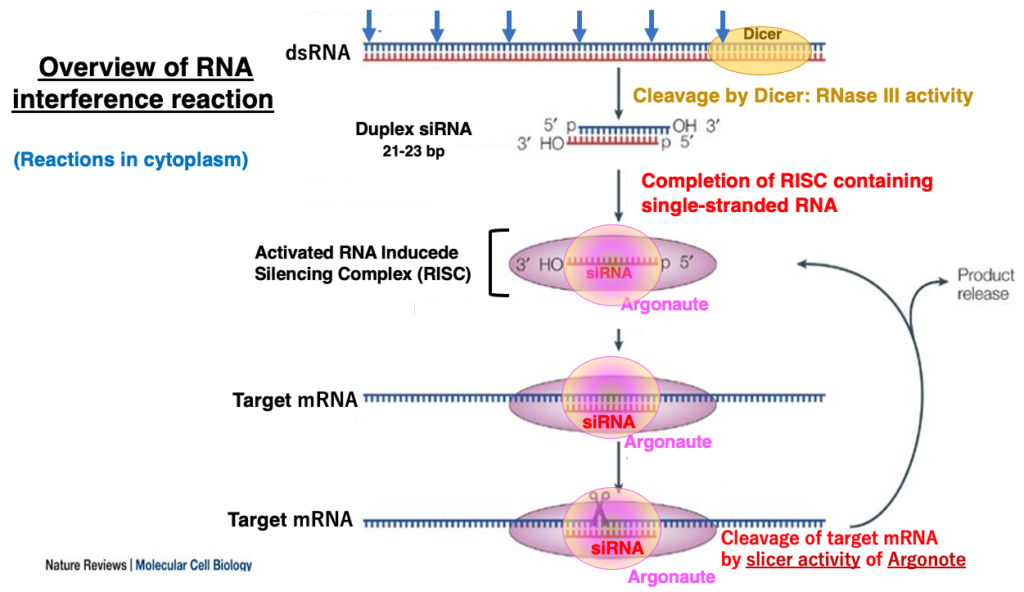
Simplified RNA interference (RNAi) reaction
First, I will explain the overview of the RNA interference reaction. Dicer, an enzyme (with RNase III activity) that specifically cleaves double-stranded RNA, cuts perfectly complementary double-stranded RNA molecules into small double-stranded RNA fragments of 21-23 nucleotides in length, in a dice-like pattern (hence the name Dicer).
These small double-stranded RNA fragments are called short interfering RNA (siRNA). They have a monophosphate at the 5′ end and a 2-nucleotide overhang at the 3′-OH end. One of the strands of this double-stranded RNA is then transferred to the RNA-Induced Silencing Complex (RISC), which contains Argonaute as its key protein.
8. Generation of duplex siRNAs
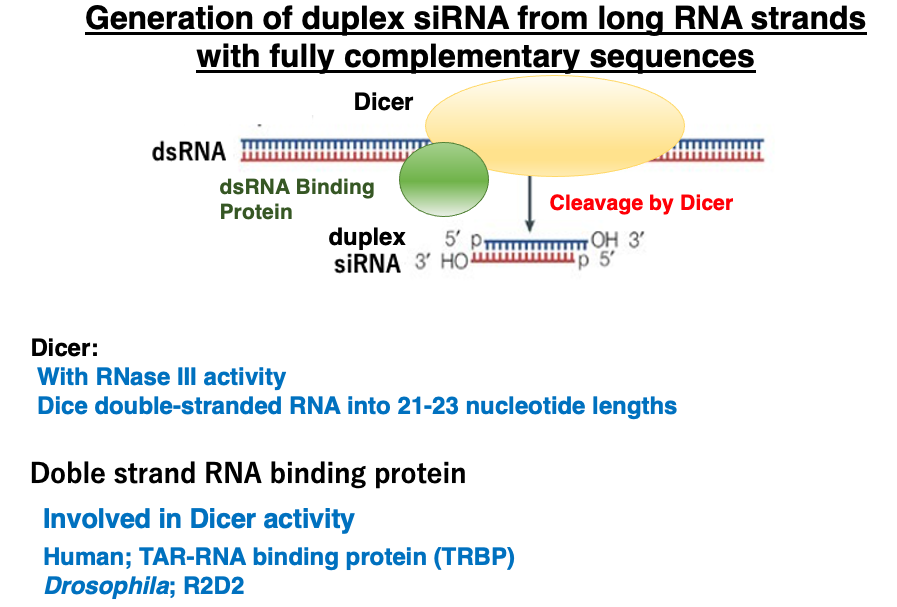
Let’s take a closer look at the cleavage of long complementary RNA by Dicer and the generation of duplex siRNAs. Dicer is an enzyme with RNase III activity that cuts double-stranded RNA into 21-23 base pair fragments from the ends.
Dicer forms a heterocomplex with double-stranded RNA binding proteins [in humans, TAR-RNA binding protein (TRBP); in Drosophila, R2D2] to cleave double-stranded RNA. The short RNA strands produced by this cleavage are called double-stranded siRNAs.
9. Characteristics of double-stranded siRNA
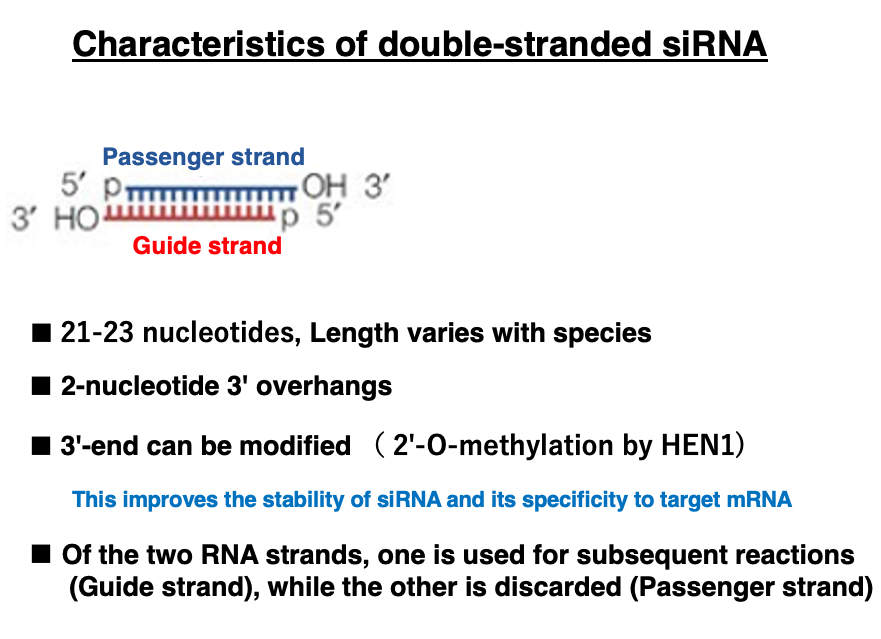
I will explain the characteristics of double-stranded siRNA. The length of double-stranded siRNA varies slightly depending on the species, but it is typically around 21-23 nucleotides long. It has a 2-nucleotide overhang at the 3′ end. Additionally, the nucleotides at the 3′ end may undergo modifications such as 2′-O-methylation. This modification is thought to improve the stability of siRNA and its specificity for target mRNA.
Of the two strands, the one that remains in Argonaute is called the Guide strand, while the one that is discarded is called the Passenger strand.
10. Loading of siRNA duplexes into Ago and release of the passenger strand
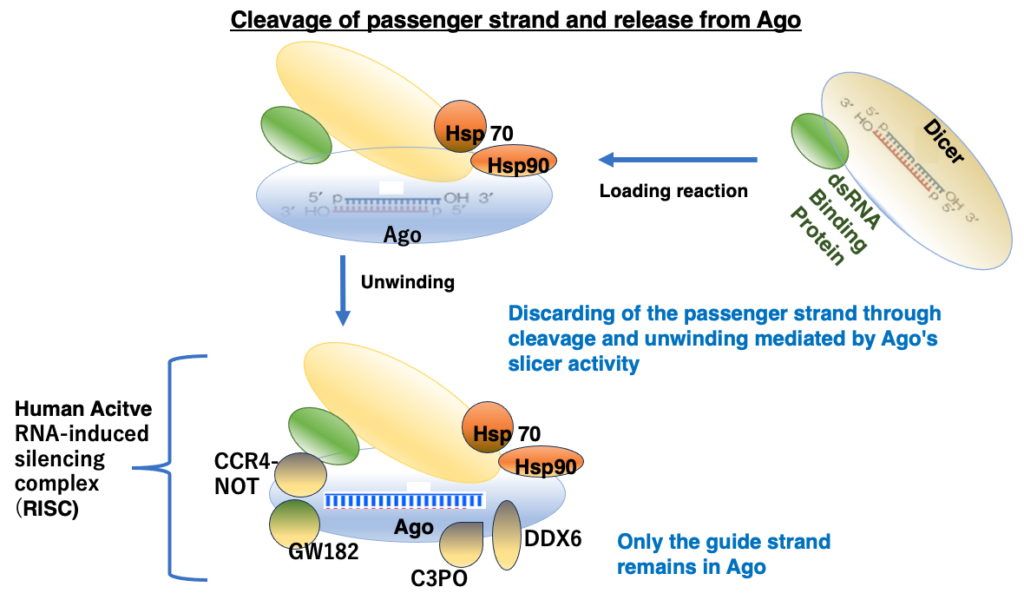
The double-stranded RNA in Dicer is transferred to Argonaute. This process requires chaperone proteins such as Hsp70 and Hsp90. Of the two strands, one is cleaved and released from Argonaute, while the uncleaved strand remains. This remaining siRNA strand is called the guide strand. Which strand is cleaved is determined by the positioning of the double-stranded siRNA when it is transferred into Argonaute.
The guide strand remains within Argonaute, and with the attachment of several additional proteins, the active RNA-Induced Silencing Complex (RISC) is completed.
11. A three-dimensional model of the complex formed by the association of Dicer, dsRNA-binding protein, and Ago
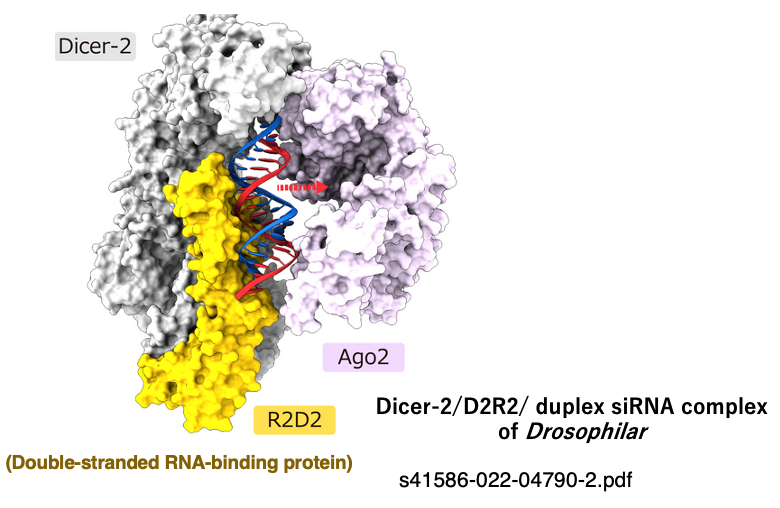
This figure illustrates the process in Drosophila where a double-stranded siRNA is about to be transferred from the Dicer-2/double-stranded RNA-binding protein (R2D2) complex to Ago-2.
12. Principles of Guide Strand Selection
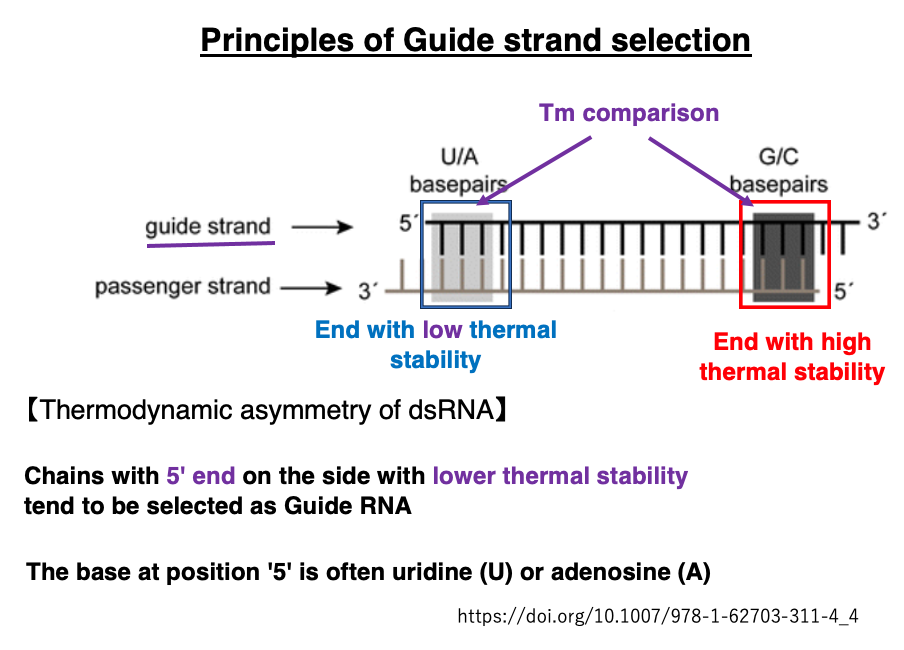
This section explains how the Guide strand, which ultimately remains within Argonaute, is determined from the double-stranded siRNA. The thermal stability (Tm) near the two ends of the double-stranded siRNA is compared. It has been found that the strand with its 5′-end on the side with lower thermal stability tends to be selected as the Guide strand.
Principles of Guide Strand Selection
This section explains how the Guide strand, which remains within Argonaute, is determined from the double-stranded siRNA. The thermal stability (Tm) near both ends of the double-stranded siRNA is compared. It has been found that the strand with its 5′-end on the side with lower thermal stability tends to be selected as the Guide strand.
13. Domains in AGO and three-dimensional structural model
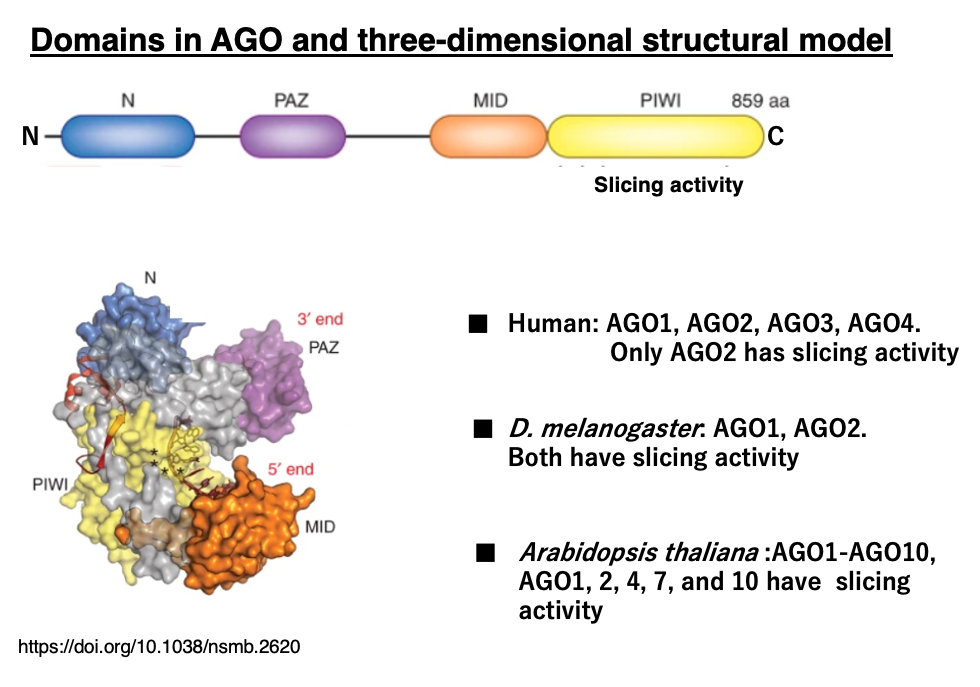
Argonaute proteins consist of several domains, with the PIWI domain near the C-terminus containing the slicer activity that cleaves mRNA or passenger strands. There are four Argonaute genes in humans, two in Drosophila, and ten in Arabidopsis. In some species, only certain Argonaute proteins possess slicer activity, and they share roles with ones that are lacking slicer activity.
14. Generation of long double-stranded RNA that can be cleaved by Dicer
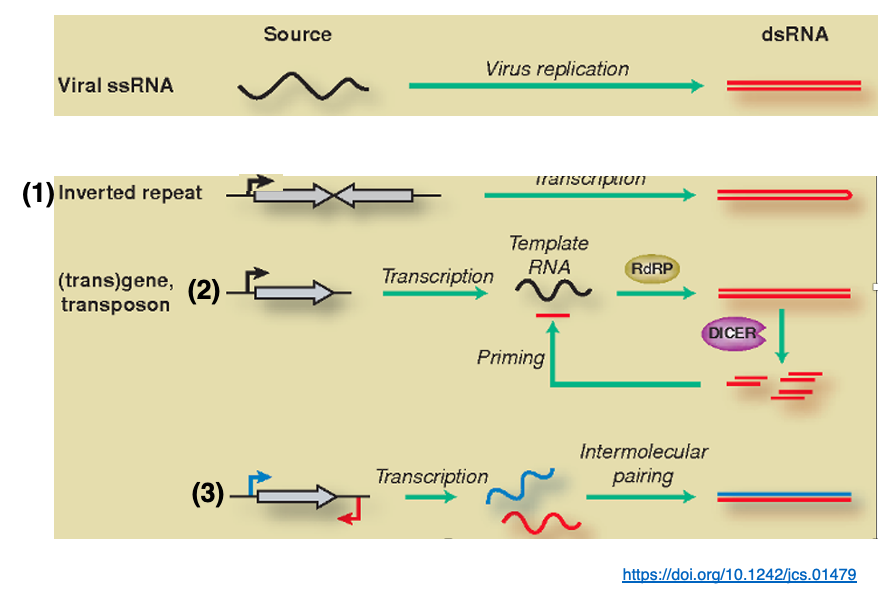
n addition to those derived from RNA viruses, other sources of RNA strands with long complementary sequences include
1. transcripts of genes with inverted repeat structures,
2. transcripts of genes or transposon mRNA that are double-stranded by RNA dependent RNA polymerase,
3. transcripts of genes in which both sense and the anti-sense are transcribed.
15. Cleavage of target mRNA by activated RISC
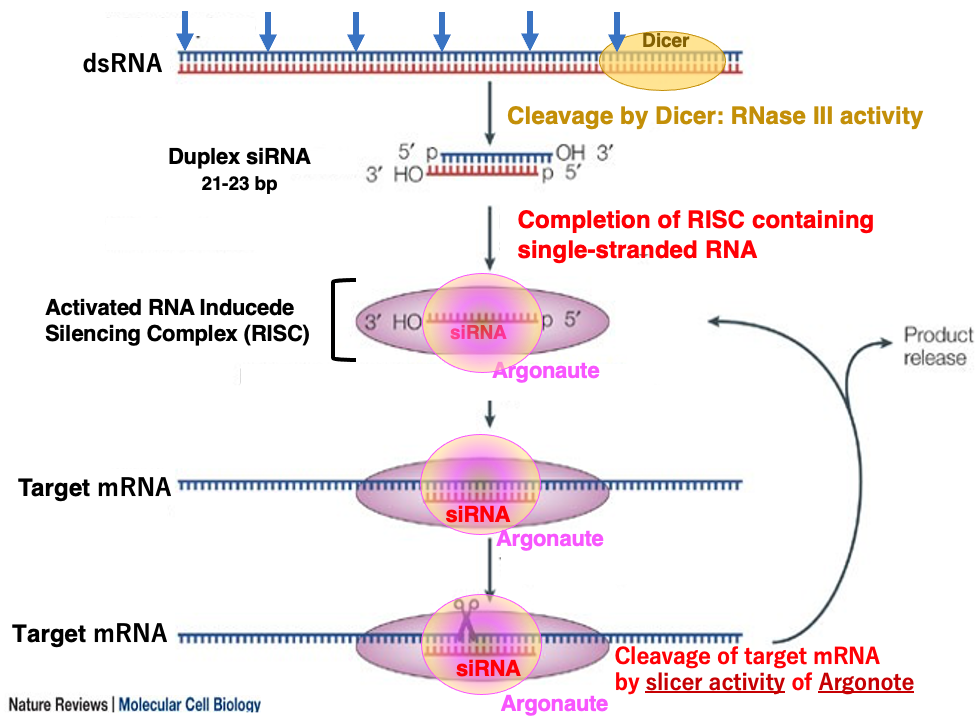
Let’s explain the reaction in which the RISC, activated by incorporation of single-stranded siRNA, searches for and cleaves the target mRNA.
16. Scanning search for target mRNA using the seed sequence of siRNA
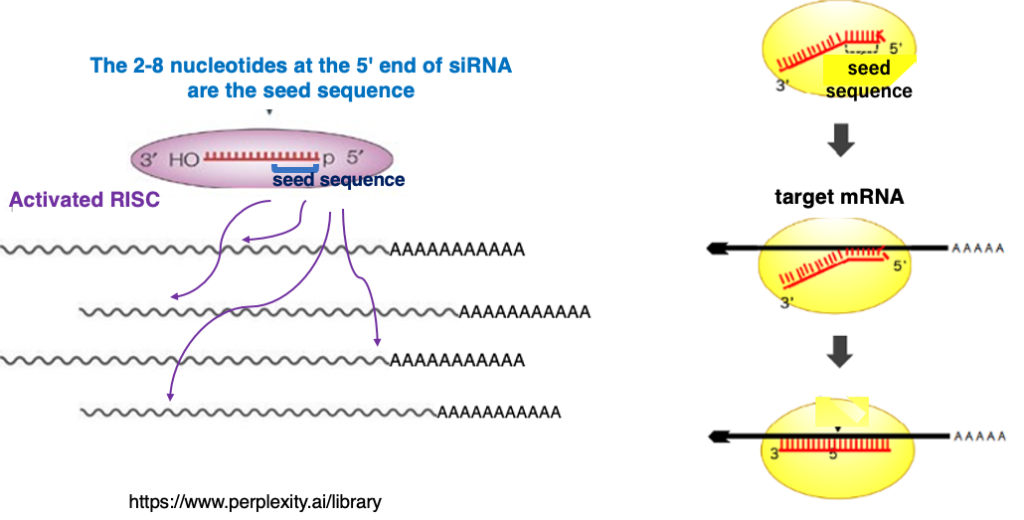
I will explain how activated RISC explore the target mRNA. The second to eighth nucleotides at the 5′ end of siRNA are referred to as the seed sequence. RISC checks whether there is complementarity with this seed sequence against the mRNA. As a result, if there is no complementarity, the scan is terminated at that point, and RISC moves on to search for the next mRNA.
Complementarity checks will continue only when seed sequences and complementarity are recognized. If entire complementarity between the siRNA and the target mRNA is confirmed, Ago within the RISC cleaves the target.
17. Search for target mRNA using the seed sequence
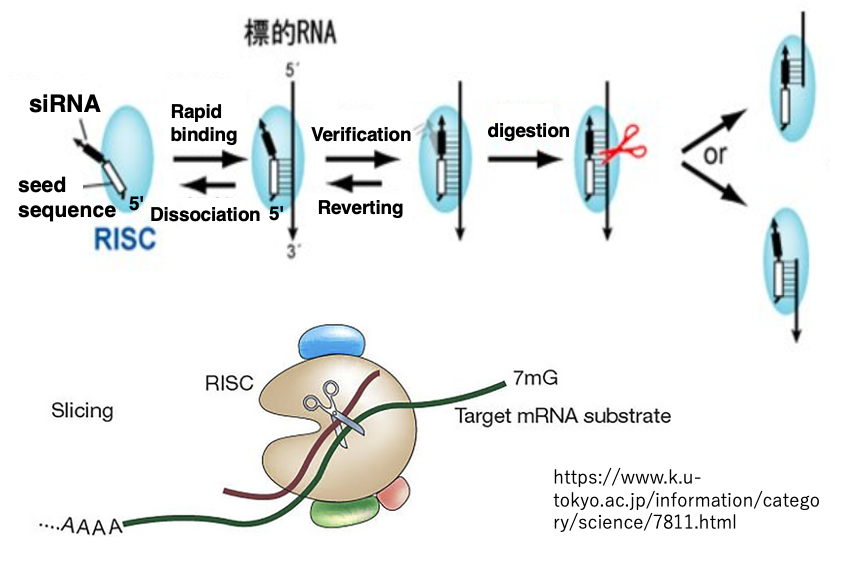
RISC will check the complementarity using the seed sequence near the 5′ end of the siRNA. At this stage, if there is no complementarity, RISC will dissociate from the mRNA. If there is complementarity, RISC will verify the complementarity for the remaining sequences.
18. Target mRNA Cleavage or Translation Inhibition by RISC
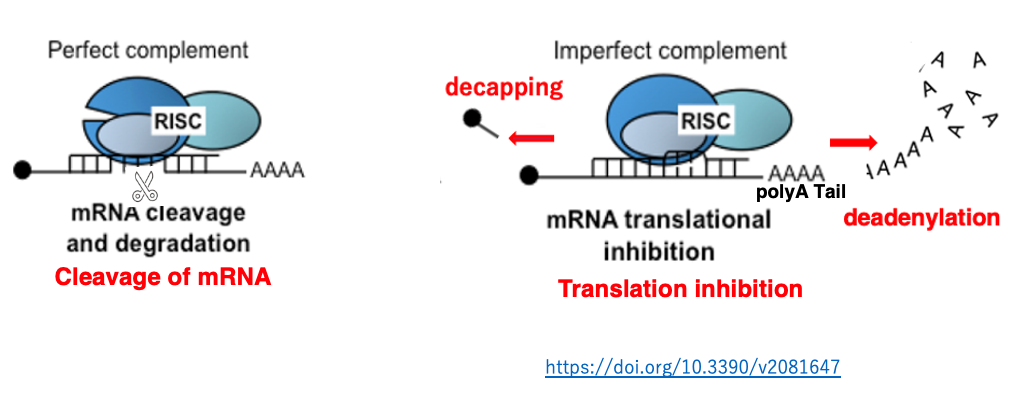
When there is complete complementarity between siRNA and mRNA, cleavage occurs. While, there is incomplete complementarity, the mRNA is not cleaved but translation is hindered.
Additionally, in the case that Argonaute does not possess slicer activity, translation suppression occurs regardless of the degree of complementarity. In such a case, degradation of the polyA or removal of the cap structure is induced, promoting mRNA degradation.
19. Mechanism of secondary siRNA production
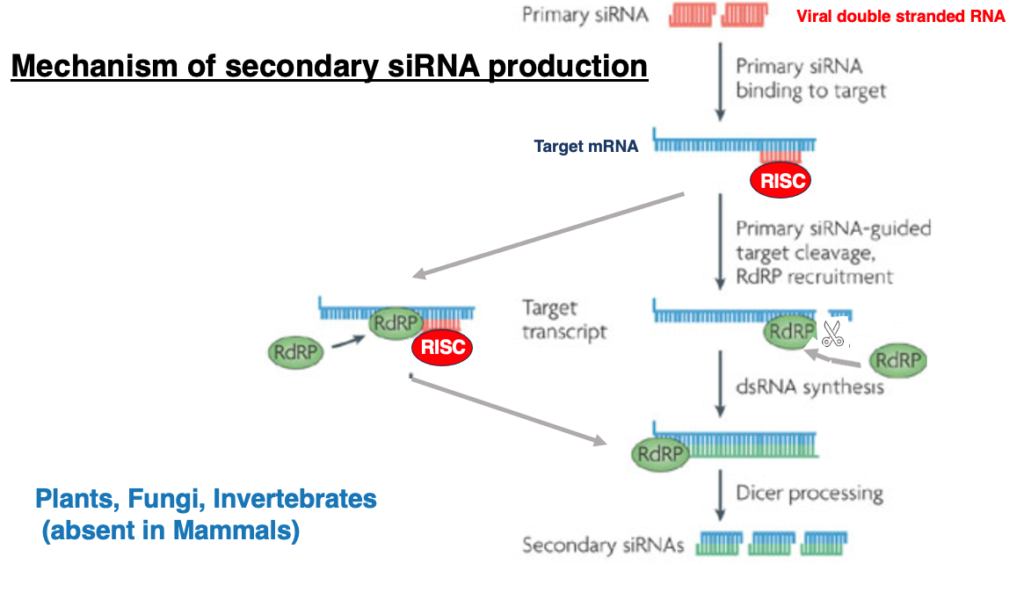
siRNA produced by cleavage of viral double-stranded RNA is referred to as primary siRNA. In addition to this, siRNA can also be produced secondarily.
Let me explain this process. In this case, even when the activated RISC binds to the target mRNA, the mRNA is not cleaved. Instead, RNA-dependent RNA polymerase is recruited, leading to the formation of double-stranded RNA from the target mRNA. Then, generated double-stranded RNA is cleaved by Dicer to produce siRNA, which is called secondary siRNA.
20. Mechanism of post-transcriptional gene silencing by miRNA
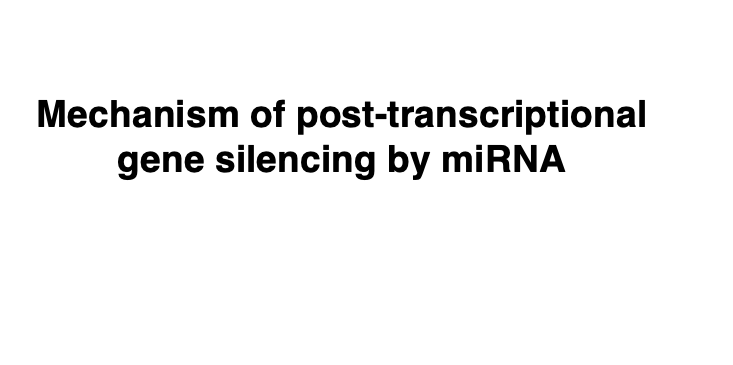
Next, I will explain the mechanism of post-transcriptional gene silencing by microRNA (miRNA).
21. Post-transcriptional gene silencing by microRNA
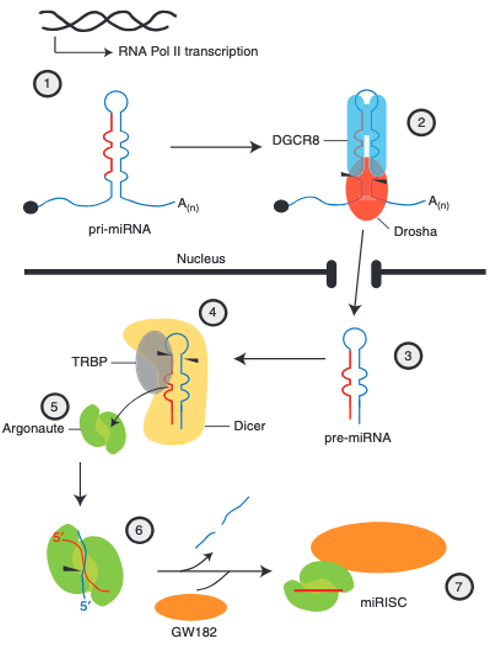
In this case, the microRNA incorporated into Argonaute is not produced from a long double-stranded RNA, but rather from a hairpin-shaped RNA that is transcribed by RNA polymerase II. The hairpin-shaped RNA in the nucleus is called primary micro RNA (pri-microRNA, pri-miRNA). The stem portion of pri-microRNA contains some mismatches.
Within the nucleus, DGCR8 recognizes the hairpin structure of pri-microRNA, attracting Drosha to form the microprocessor complex. Drosha trims the 5′ and 3′ ends of the pri-microRNA. This processed hairpin RNA is referred to as precursor micro RNA (pre-microRNA, pre-miRNA). Pre-microRNA is then transported from the nucleus to the cytoplasm.
In the cytoplasm, Dicer and TRBP attach to pre-microRNA, removing parts such as the hairpin structure, resulting in a double-stranded RNA of 19-25 nucleotides (duplex microRNA). This double-stranded microRNA is handed over to Argonaute protein, where only the guide strand remains. When proteins such as GW182 bind to Argonaute containing the guide strand, Argonaute becomes activated.
22. Precursor microRNA and mature microRNA of Drosophila
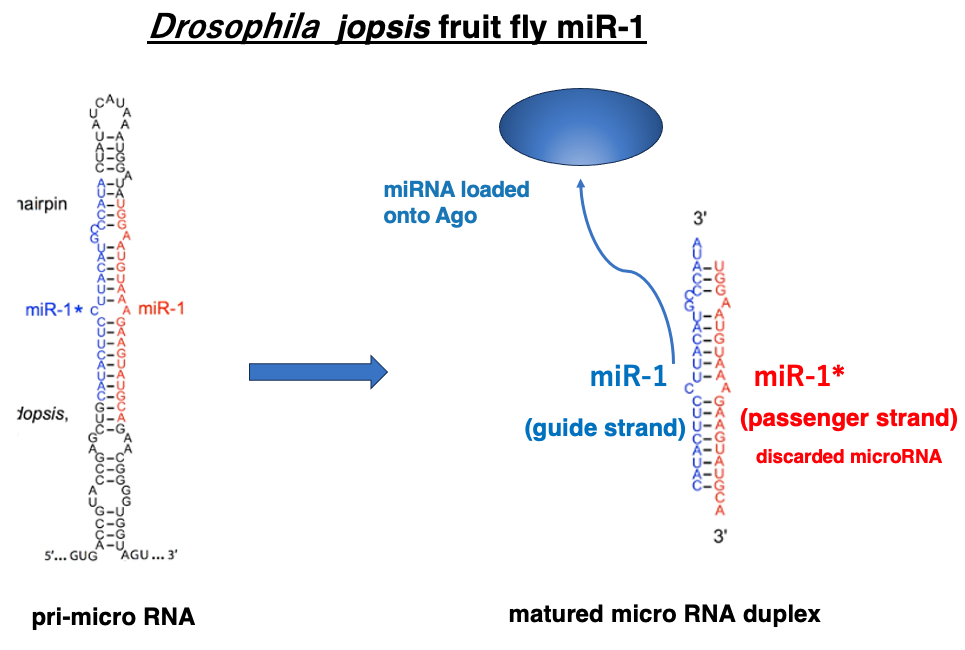
This figure shows one of the microRNAs in Drosophila, miR-1. The left side of the figure depicts the pri-microRNA immediately after transcription in the nucleus, while the right side shows the mature double-stranded microRNA.
Similar to siRNA, the 3′ end protrudes by two nucleotides. In this figure, the blue-lettered sequence represents the guide strand, which remains within Argonaute. The mechanism for selecting the guide strand from the double-stranded microRNA is the same as in the case of siRNA. It based on differences in thermal stability at the ends of the sequences.
23. The pathway of Post-Transcriptional Gene Silencing by miRNA
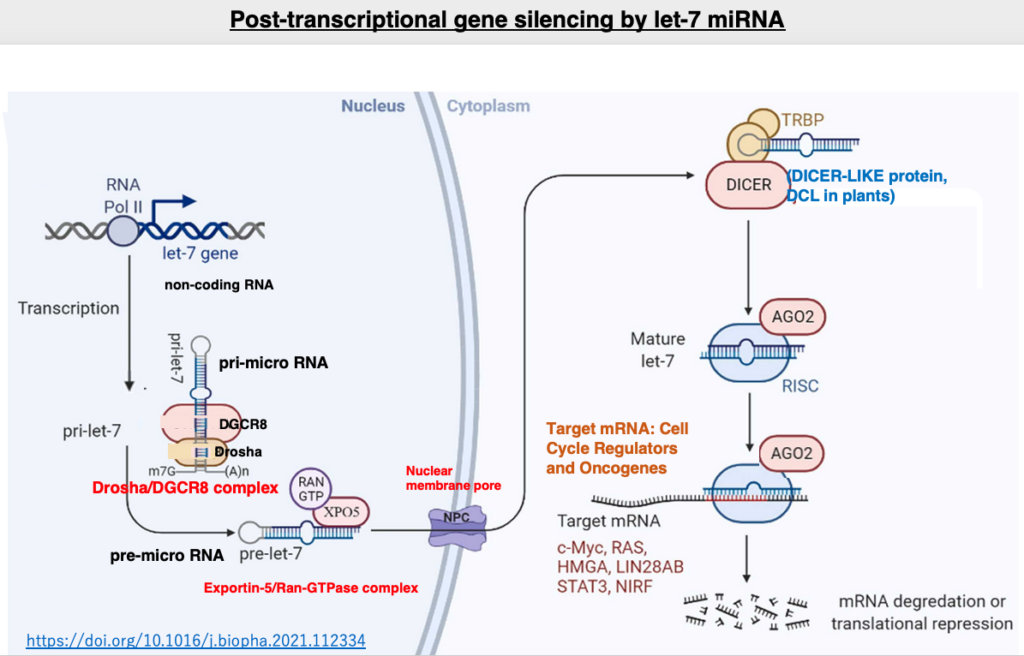
Let’s examine the post-transcriptional suppression by let-7 microRNA, which is known to be involved in the development of human hematological cancers. The let-7 gene is transcribed by RNA polymerase II. The transcribed pri-let-7 microRNA is processed by the Drosha and DGCR8 complex, which cleaves parts of the 5′ and 3′ ends to form pre-microRNA. The pre-microRNA is then bound by the Exportin-5/Ran-GTPase complex and transported through the nuclear pore to the cytoplasm.
In the cytoplasm, the double-stranded RNA binding protein TRBP and Dicer are added to the pre-microRNA, which is then processed into a 22-nucleotide double-stranded microRNA. This RNA is handed over to Argonaute, where only the guide strand remains inside. The activated RISC is formed by the single-stranded guide strand and the addition of other proteins.
RISC searches for mRNAs with high complementarity to the guide strand in the cytoplasm. The base complementarity between the guide strand and the target mRNA does not need to be 100% matching, and 2-3 base mismatches are tolerated. The binding of RISC inhibits the translation of the target mRNA while also promoting its degradation. It is now known that the targets of let-7 microRNA are not singular, but include multiple mRNAs encoding c-Myc, RAS, HMGA, and so on.
24. Comparison of post-transcriptional repression by siRNA and miRNA
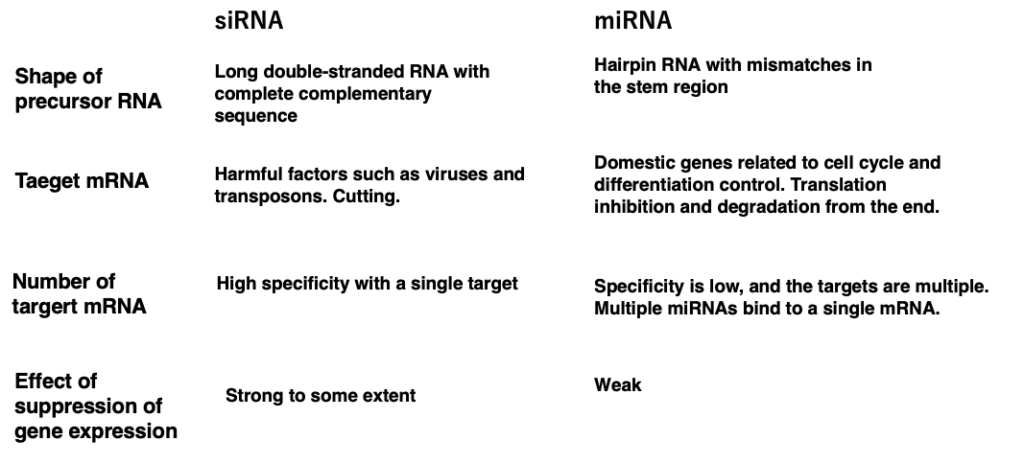
This table summarizes the differences between siRNA and miRNA. First, let’s consider the differences in the precursor RNAs from which siRNAs and miRNAs are derived.
1. siRNAs are excised from long double-stranded RNAs with perfect complementarity, whereas miRNAs are cut out from hairpin RNAs that contain mismatches in the stem region.
2. The target mRNAs of siRNAs are typically derived from harmful factors such as viruses and transposons, and these target RNAs are cleaved. On the other hand, miRNA targets are usually endogenous mRNAs involved in cell cycle and differentiation control. miRNAs induce translational inhibition and degradation from the ends of the target mRNAs through RISC attachment.
While siRNA targets a single molecule, miRNA can have multiple target mRNAs. Additionally, the gene expression suppression effect of siRNA is relatively strong, but the effect of miRNA is not as potent. If artificial siRNA or miRNA can be introduced into the cytoplasm of cells, it may be possible to simultaneously suppress the expression of specific genes or groups of genes. However, the degree of effectiveness of siRNA and miRNA seems to vary greatly depending on the species.
25. Comparison of gene silencing pathways by siRNA and miRNA
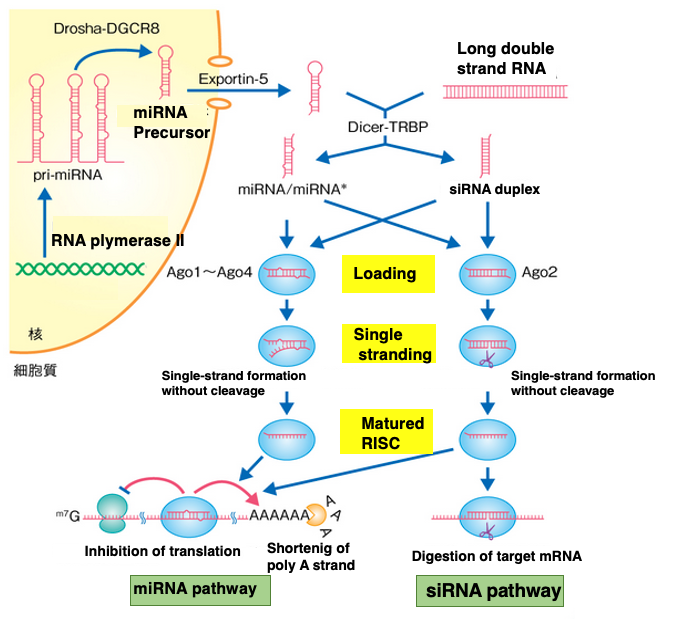
This figure simultaneously illustrates the pathways for gene silencing by siRNA and miRNA.
In these two pathways, it can be observed that the Dicer-TRBP complex, Ago, and RISC are not specialized for either pathway, but rather the same proteins are utilized in both pathways.
26. Long-distance movement of siRNA in plants
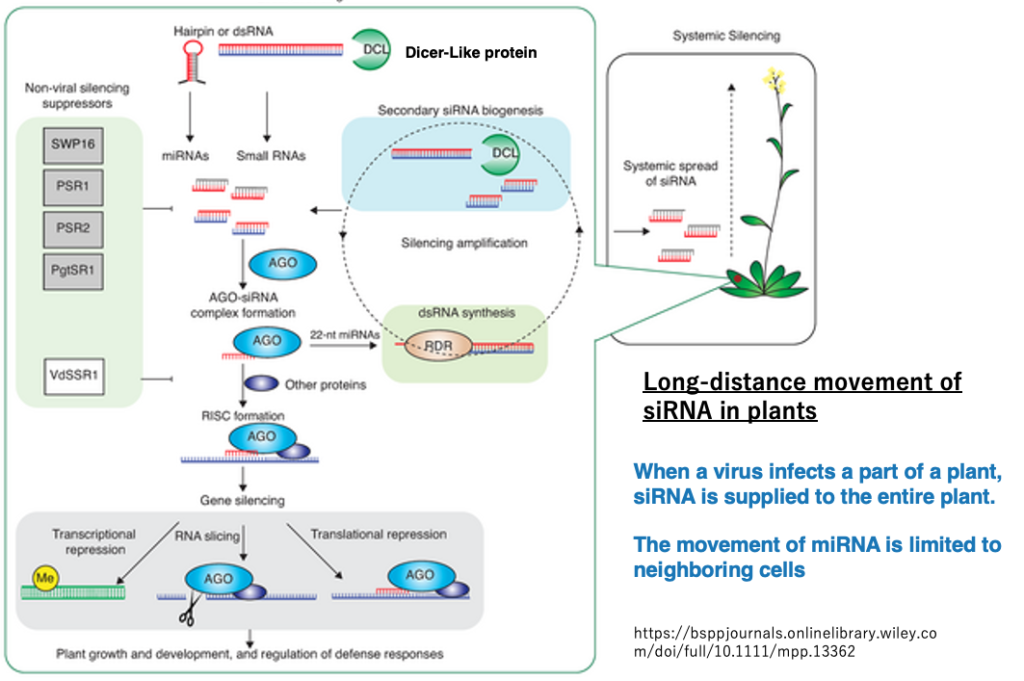
In land plants, when a virus infects some leaves and siRNA is produced, it is known that the virus defense system spreads throughout the entire plant body as siRNA moves through the phloem.
On the other hand, in the case of miRNA, its movement is limited to spreading only to surrounding cells.
27. RNA silencing suppressors against plant viruses
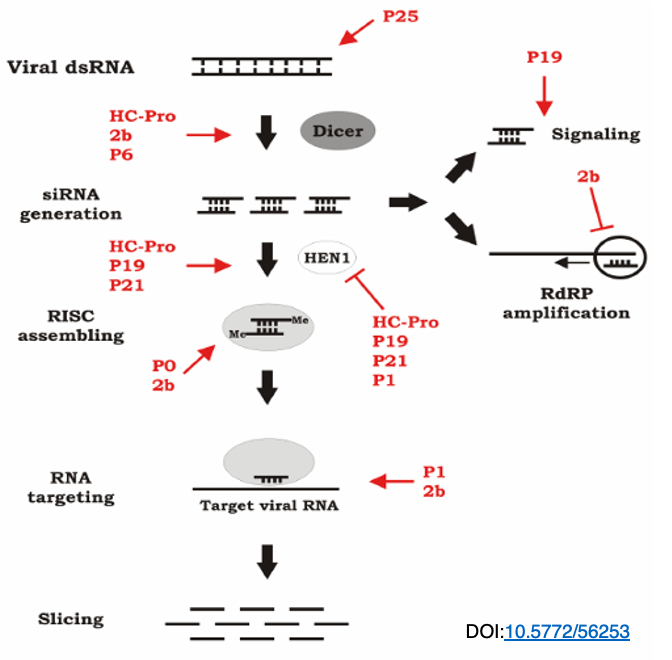
RNA interference is a defense mechanism that hosts have against viruses, but viruses also counteract this by expressing proteins that interfere with the host’s RNA interference. In this figure, the viral proteins that interfere with the RNA interference reaction are shown in red.
As illustrated, viruses possess various proteins that interfere with different steps of RNA interference, thus countering the host’s inhibition of viral replication through RNA interference.
28. Examples of RNA silencing suppressors
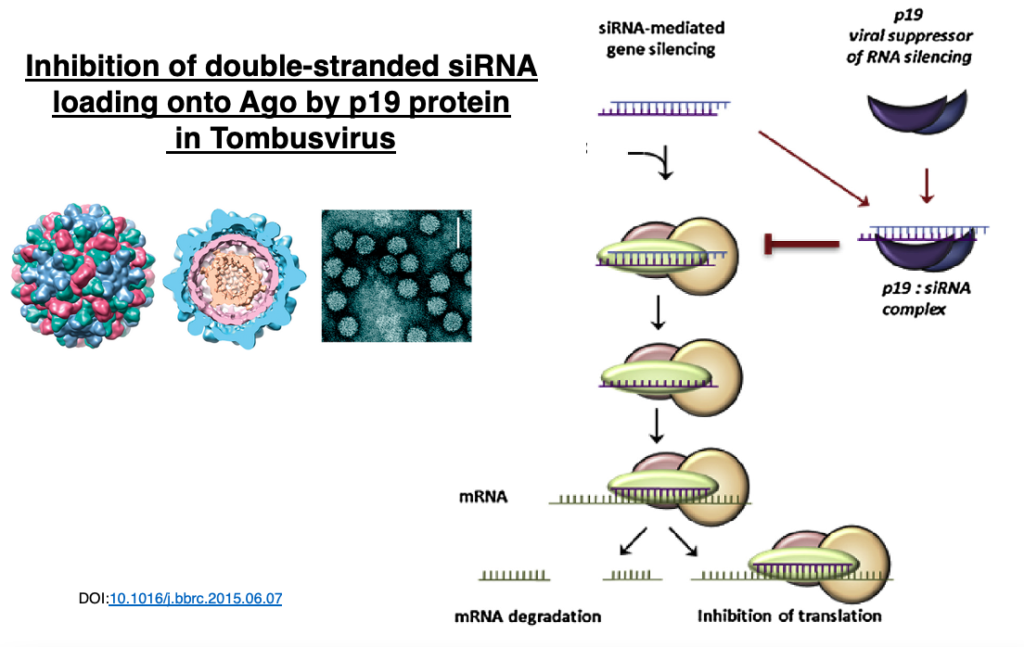
In plant viruses of the genus Tombusvirus, the p19 protein dimer attaches to siRNA duplexes, inhibiting their loading onto Argonaute.
This interferes with the plant’s RNA interference mechanism.
29. Propagation of miRNA via Exosomes
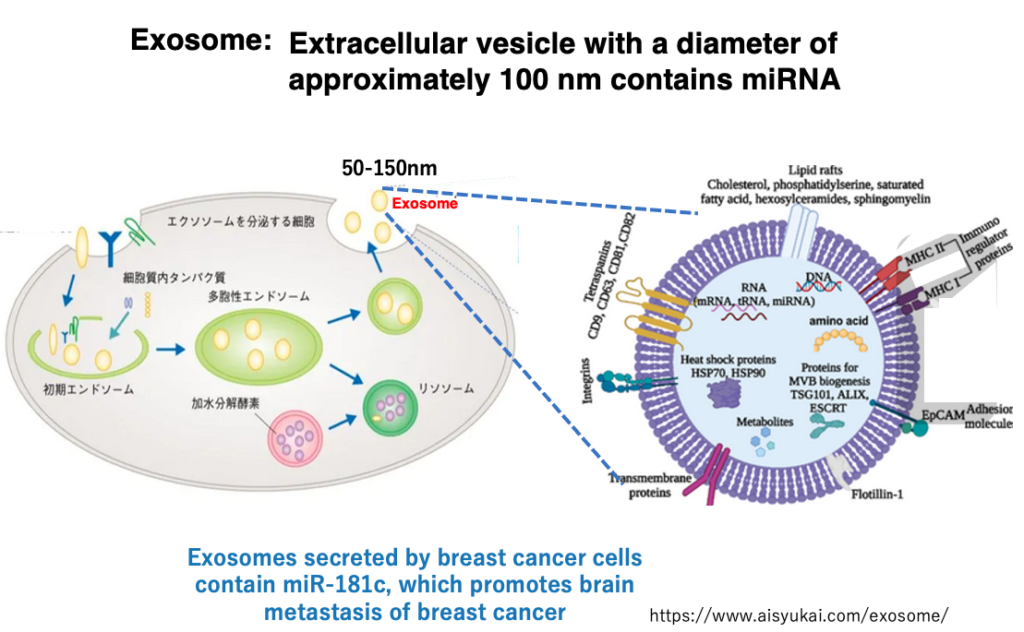
In animals, while siRNA does not move to distant cells, miRNA can be transferred to cells far away. Long-distance intercellular communication is achieved through secretory lipid bilayer vesicles called ‘exosomes’, which are approximately 100 nm in diameter.
These exosomes are secreted from cells and transported throughout the body via blood and lymph fluid. Exosomes contain various proteins and nucleic acids, including miRNAs.
For example, exosomes secreted by breast cancer cells contain a type of microRNA called miR-181c, which has been found to promote the metastasis of breast cancer to the brain.
30. Mechanism of post-transcriptional gene silencing by piRNAs
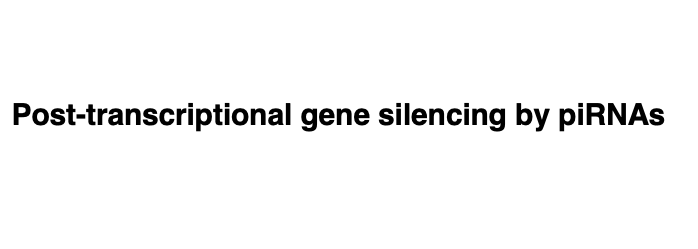
Next, I will explain the mechanism of post-transcriptional gene silencing by PIWI-interacting RNA (piRNA). This RNA interference by piRNA occurs only in animal and insect germ cells and contributes to the suppression of transposon movement in germ cells.
Genomic mutations in germ cells affect the entire organism and can also be a driving force for evolution. piRNAs have not been found in plants. It is known that when RNA interference by piRNA does not function normally, infertility can be caused, or frequent genomic DNA cleavage occurs in germ cells.
31. Comparing piRNAs and miRNAs
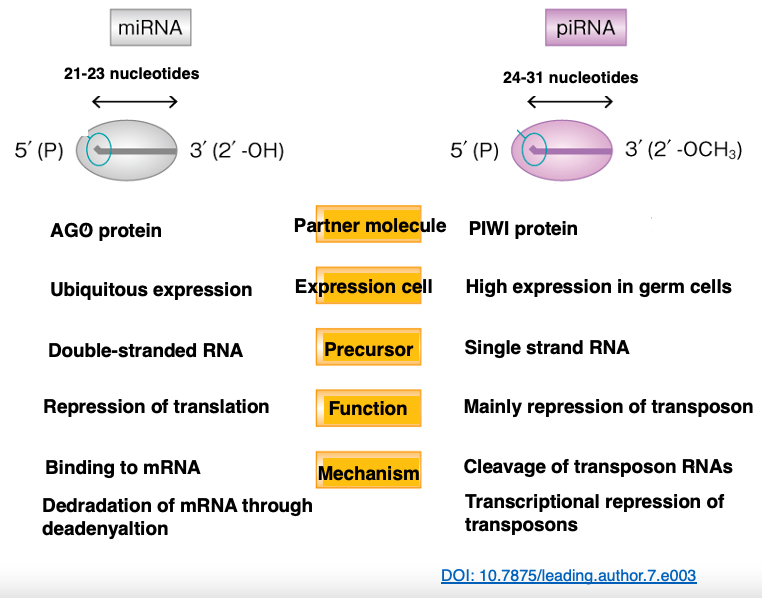
Let’s compare the lengths of piRNA and miRNA. piRNAs tend to be longer, with 24-31 nucleotides, compared to miRNAs which are 21-23 nucleotides long. Additionally, while the partner protein for miRNAs is the Argonaute protein, for piRNAs, it’s the PIWI protein.
miRNAs are expressed in all cells, but piRNAs are highly expressed only in germ cells. The precursor of miRNAs is hairpin RNA, whereas the precursor of piRNAs is a long single-stranded RNA. miRNAs target various mRNAs, but piRNAs are limited to targeting transposon-derived RNAs.
miRNAs bind to their target mRNAs and induce degradation, while piRNAs cleave transposon-derived RNAs.
32. Uni-strande piRNA cluster in Drosophila genome
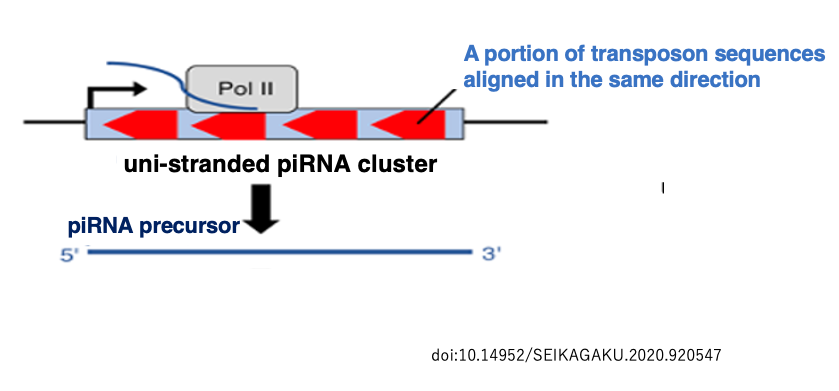
In the Drosophila genome, there are regions known as uni-strand piRNA clusters where fragments of various transposons are gathered in the same orientation with respect to the sense and antisense strands.
One of these, called flamenco, spans up to 180 kb. Flamenco is transcribed by RNA polymerase II. The resulting RNA transcript contains fragments of various transposons in the antisense orientation. piRNAs are produced from this type of RNA.
33. Generation pathway of activated Piwi-piRSC
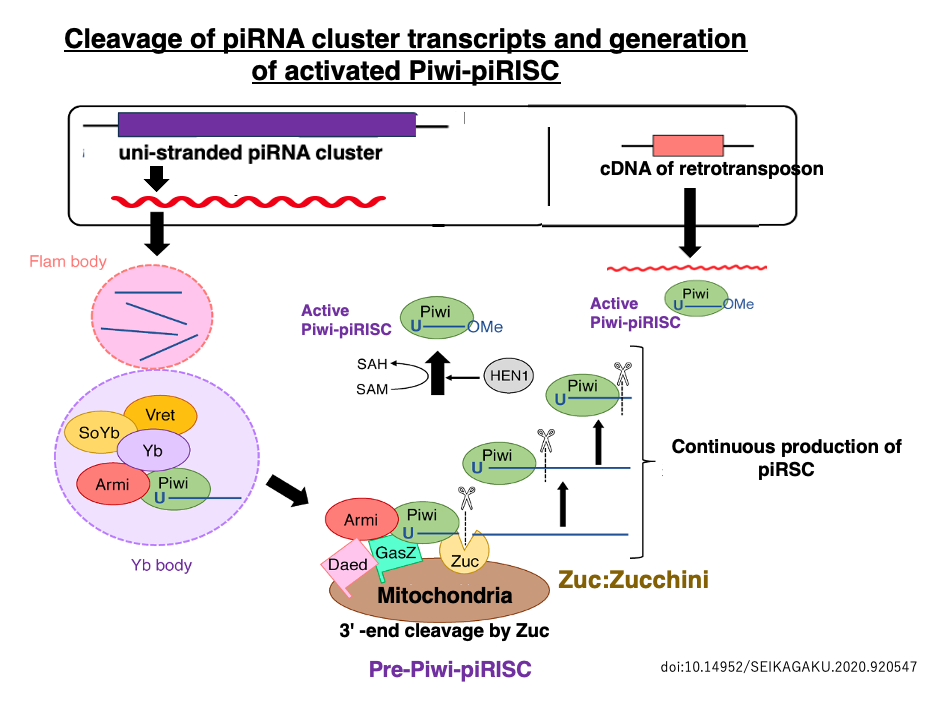
This long single-stranded RNA accumulates in the Flam body and then moves to the Yb body, where it undergoes rough cleavage. This Pre-Piwi RNA binds to PIWI, a protein in the same family as Argonaute, forming the Pre-Piwi-piRISC.
The endoribonuclease Zucchini (Zuc), located on the mitochondrial membrane, cleaves the ends of the Pre-piRNA within Piwi, resulting in the formation of mature piRNAs 24-31 nucleotides in length inside Piwi. This process gives rise to the mature Piwi-piRISC.
The remaining long flamenco RNA (piRNA precursor) is captured by another PIWI protein and, similar to the previous process, its 3′ end is cleaved by Zuc in a Dicer-independent reaction that repeats.
As a result, a large number of mature piRNAs are produced from a single-stranded piRNA precursor, leading to the production of active Piwi-piRSC. The activated Piwi-piRISC searches for transposon-derived RNAs with complementarity to the piRNA in the cytoplasm. When it finds such RNAs, it either cleaves them or induces translational repression.
34. The ping-pong pathway for producing mature piRNAs from dual-strand cluster transcripts
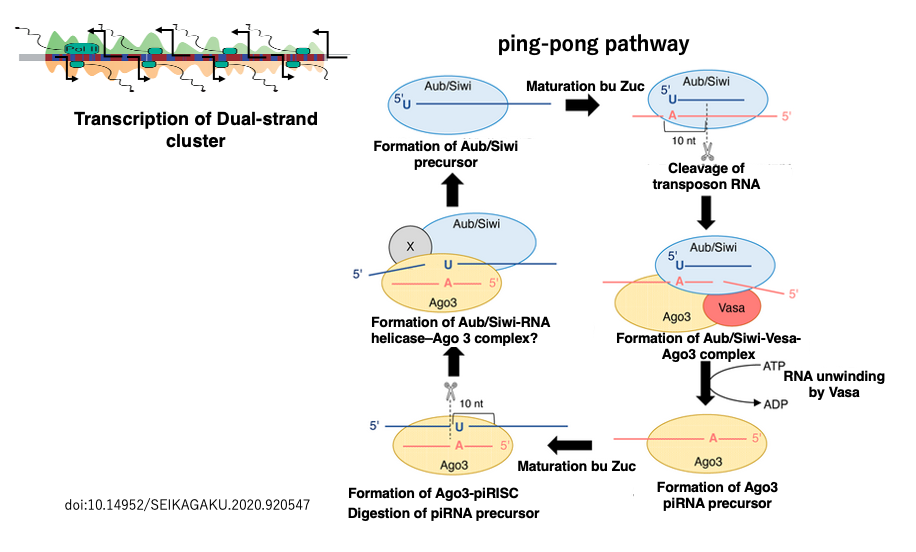
In Drosophila, the transposon fragments encoded in the flamenco region have directionality, and their transcripts become antisense sequences of transposons, making them suitable as piRNA precursors. On the other hand, in the heterochromatin regions near the centromeres and telomeres of Drosophila, there are areas where transposon fragments are encoded in both sense and antisense orientations (such as 42AB, 38C, 80F).
These regions are called dual-strand clusters because transcription occurs from both strands of the genome. The transcripts from both strands of these regions are used in a way where piRNAs produced from one strand are used to generate piRNAs from the transcript of the other strand.
Therefore, this piRNA production pathway is called the ping-pong pathway. The process by which piRNAs become incorporated into Piwi proteins to form active Piwi-piRISCs is the same as when piRNAs are produced from uni-strand piRNA clusters. Unlike siRNA and miRNA production, which involves Dicer, piRNA production does not involve Dicer.
Well then, this concludes the lecture.
コメント