This lecture can be viewed as a video on YouTube site: Life Scinence Lectures for you
https://www.youtube.com/watch?v=ppxJSdIdbZs /
1. Contents of this lecture
In this lecture, the relationship between the genetic code and transfer RNA is included. This article contains 29 figures.
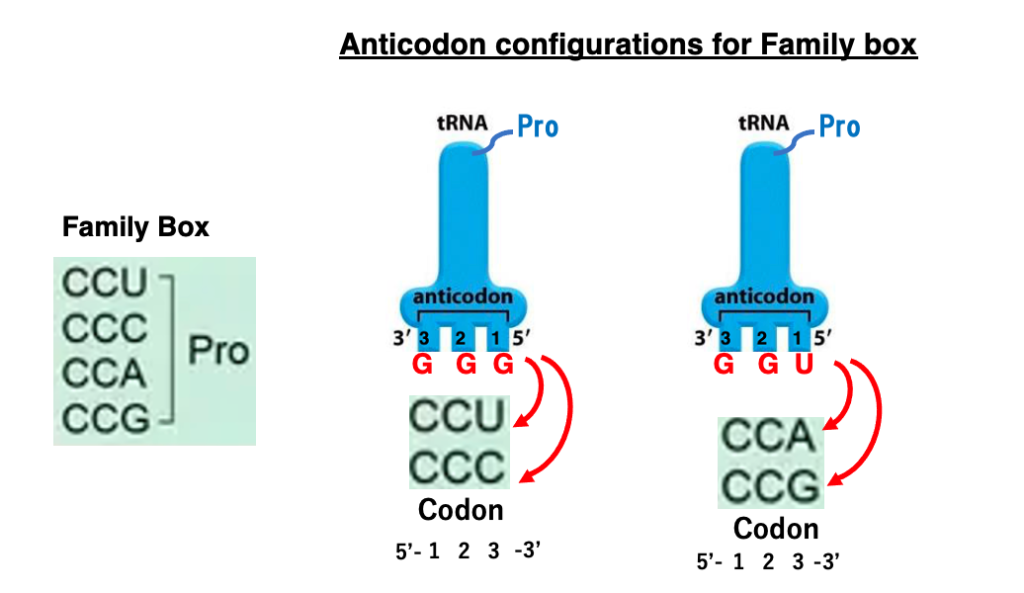
- 1. Characteristics and roles of the transfer RNA molecule: amino acid addition to the second prime hydroxyl or third prime -hydroxyl group of CCA
- 2. Two steps for generation of aminoacylated transfer RNA
- 3. Chemical conversion of amino acids accepted by transfer RNA
- 4. Two codon set and Family box
- 5. Wobble base pair rule
- 6. Release factor
- 7. Initiator transfer RNAs and Elongator transfer RNA
Key Words:
2. How amino acids are added to transfer RNAs
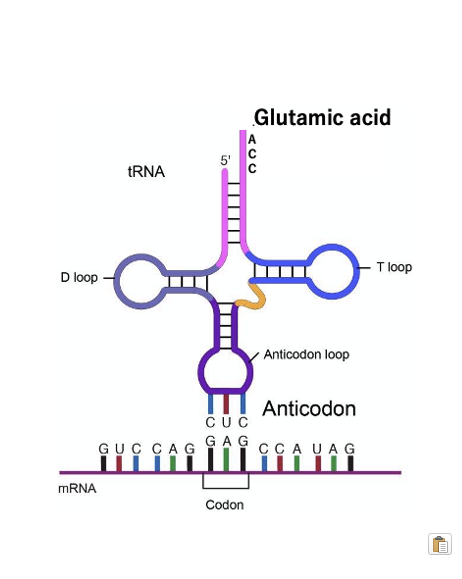
First, I will explain the mechanism how amino acids are added to transfer RNAs, and the characteristics of the genetic code table.
Here is an overview of characteristics of transfer RNA: transfer RNA is a single-stranded RNA molecule of around 80 nucleotides that carries a specific amino acid to a particular codon on the messenger RNA. Its 3′ end always terminates with the CCA sequence. Additionally, the anticodon loop region contains an anticodon that can form stable hydrogen bonds, by base-pairing with the corresponding codon on the mRNA.
This image shows a transfer RNA with the anticodon CUC carrying the amino acid glutamine attached to its 3′ end, forming stable hydrogen bonds with the GAG codon on the messenger RNA.
3. Twenty different Aminoacyl-transfer RNA synthetases (ARSs)
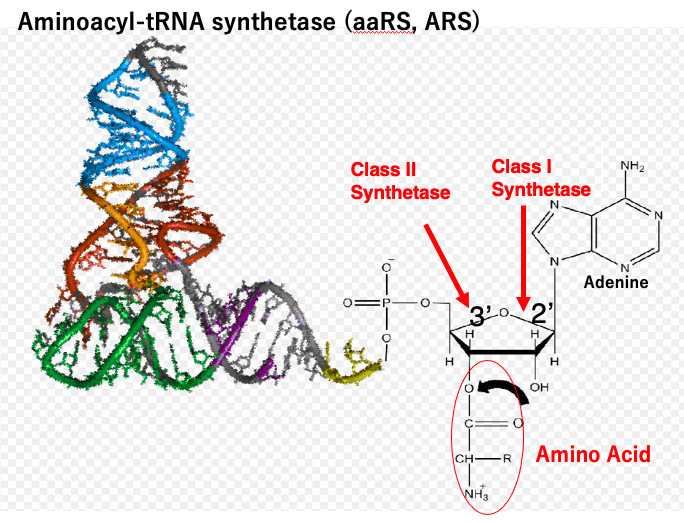
Aminoacyl-transfer RNA synthetases, abbreviated as ARSs, are enzymes that have the activity to attach specific amino acids to specific transfer RNAs.
There are 20 different amino acids used in cells, so in principle, there are 20 different ARSs. However, it is known that there are 40 to 60 different types of transfer RNAs, depending on when they are counted based on differences in their internal base sequences. ARSs are broadly classified into two groups based on the position of the ribose to which they attach the amino acid.
Class I ARSs have the activity to attach the amino acid to the second prime hydroxyl group of the ribose of the adenosine in the third prime-CCA end of the transfer RNA. On the other hand, Class II ARSs have the activity to attach the amino acid to the third prime – hydroxyl group of the adenosine. In this way, amino acids are attached to either the second prime hydroxyl group or third prime – hydroxyl group of the terminal adenosine.
4. Class I ARS and Class II ARS
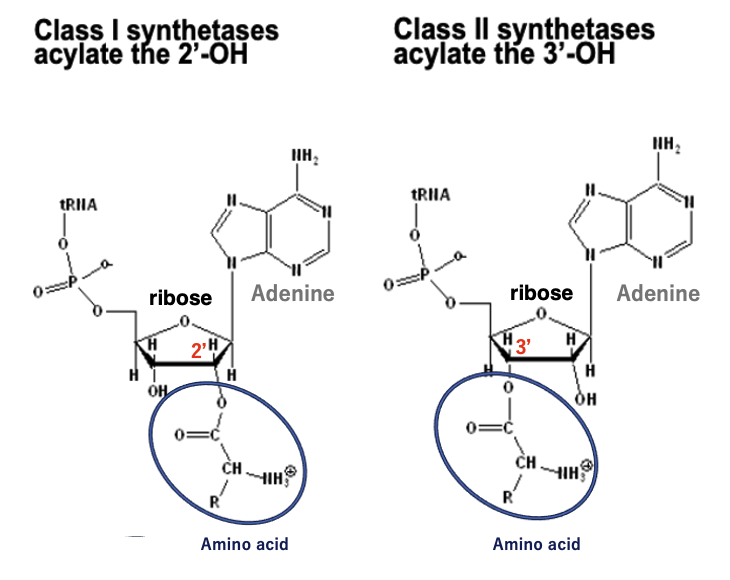
This is the summary. Class I ARS adds an amino acid at the second prime -hydroxyl group of the sugar of adenosine, while Class II ARS adds an amino acid at the third prime – hydroxyl group of the sugar.
5. Classification of ARS’s
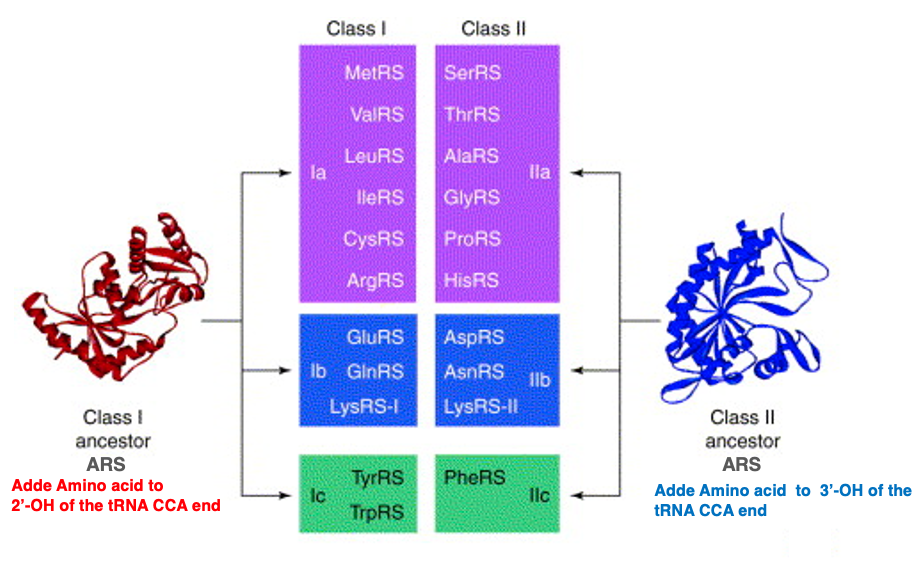
This table summarizes Class I and Class II aminoacyl-transfer RNA synthetases. The ARSs that can aminoacylate methionine- transfer RNA with methionine, as well as those that aminoacylate transfer RNAs with valine, leucine, isoleucine, cysteine, arginine, glutamic acid, glutamine, lysine, tyrosine, and tryptophan belong to Class I. In other words, they have the activity of attaching amino acids to the second prime -hydroxyl group of the ribose.
On the other hand, those listed here are Class II ARSs, which attach amino acids to the third prime – hydroxyl group of the ribose. It is known that the reaction in which an ARS recognizes a specific transfer RNA and attaches a specific amino acid occurs in two steps. Next, I will explain this reaction.
6. Formation of Aminoacyl AMP
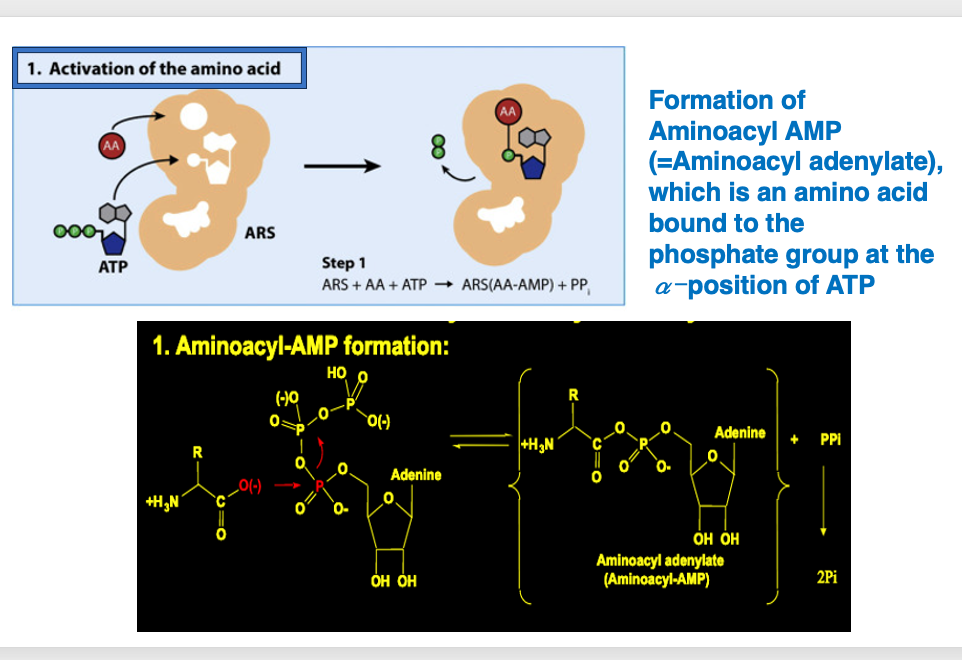
In the first reaction, ARS recognizes the amino acids to be taken up by itself, and takes them into ARS. In the ARS, an amino acid is added to the phosphate group at the α-position of ATP to form aminoacyl AMP. The chemical formula is schematically shown here: Aminoacyl AMP is formed by the addition of an amino acid to the phosphate group at the α-position of ATP, and pyrophosphate is released. This shows the chemical formula of the reaction.
7. Transfers amino acids from aminoacyl AMP to the 2′-OH or 3′-OH of the transfer RNA
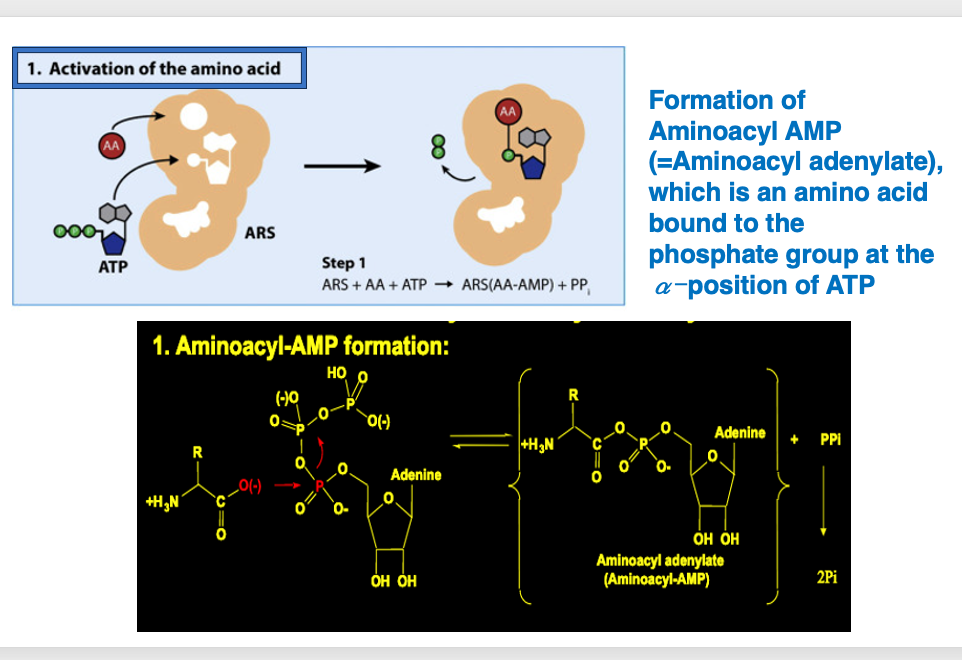
In the second step, ARS recognizes the transfer RNA to be incorporated into the enzyme, which then transfers amino acids from aminoacyl AMP to the 2′-OH or 3′-OH of the transfer RNA. The chemical equation for this reaction is shown below. Amino acid attached to the AMP is transferred to a transfer RNA, and AMP is released by the same reaction.
8. Summary of the Step 1 and Step 2
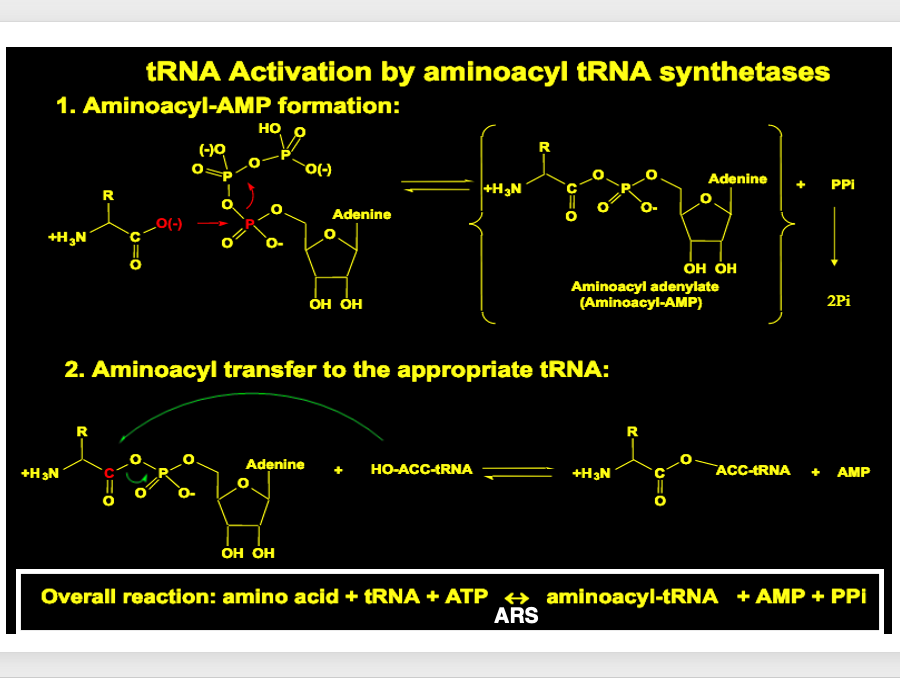
The two reactions can be summarized as follows. ARS catalyzes the reaction. Finally, a transfer RNA molecule is formed in which a specific amino acid is added to a specific transfer RNA, and AMP and pyrophosphate are released.
9. Identity of tRNAs
There are multiple types of tRNAs that carry Leucine (Leu), and their internal base sequences are different.However, there is only one type of Aminoacyl-tRNA Synthetase (ARS) that attaches Leu to tRNA. So how does the Leu-ARS distinguish the Leu-tRNAs from other tRNAs?
10. How ARSs recognize transfer RNAs
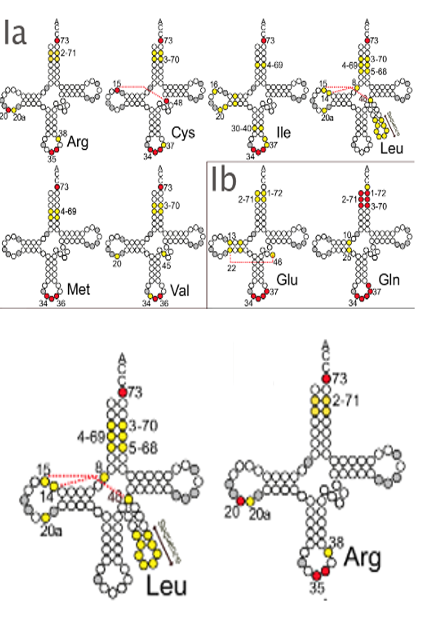
I will explain how ARSs recognize transfer RNAs. For example, there are multiple types of transfer RNAs that carry leucine within the cell, and their internal base sequences are not the same. On the other hand, there is only one type of ARS that adds leucine to transfer RNA. Therefore, the question arises as to how the leucine ARS distinguishes the leucine transfer RNA from transfer RNAs that accept amino acids other than leucine. Here is a diagram of the leucine transfer RNA, but it is known that the 73rd base is always a specific base common to all transfer RNAs that carry leucine. In addition, the bases colored in yellow are highly conserved among leucine transfer RNAs. Such bases are called identity bases that determine the identity of the transfer RNA. For example, this is an arginine transfer RNA, and it is known that bases such as the 73rd, 20th, 35th, and 6th are common among arginine transfer RNAs and are used as identity bases. ARSs strongly recognize the identity bases of each transfer RNA, thereby recognizing which transfer RNA carries which amino acid.
11. Chemical modifications of tRNA-accepting amino acids
Some bacteria have aspartic acid transfer RNA synthetase but lack asparagine transfer RNA synthetase. Similarly, they have glutamic acid transfer RNA synthetase but lack glutamine transfer RNA synthetase. So, how asparagine accepted transfer RNA and glutamine accepted transfer RNA are generated. The solution is the amino acid conversion on transfer RNA.
12. Chemical modifications of tRNA-accepting amino acids:Asp to Asn and Glu to Gln
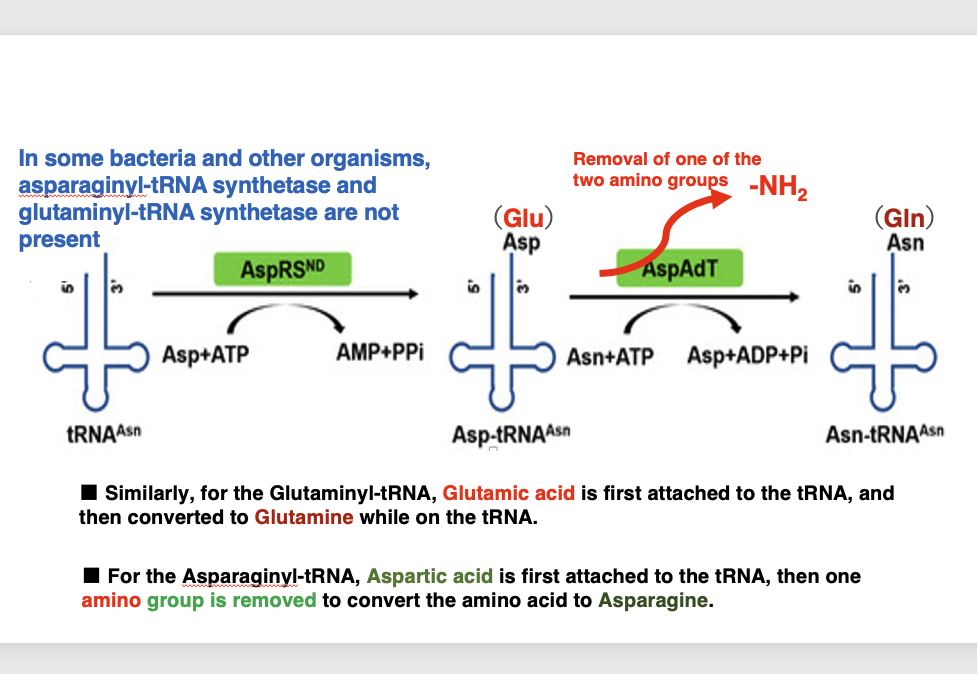
We will discuss examples of amino acids being converted to other amino acids by chemical modifications on the accepted tRNA. It is known that in some bacteria, glutamate ARS is present but glutamine ARS is absent, or aspartate ARS is present but aspartate ARS is absent. In these cases, the amino acid is converted on the tRNA.
Aspartic acid is converted to asparagine by removing one amino group from aspartic acid. This creates asparagine-accepting asparagine-tRNA. Similarly, some bacteria do not have glutamine ARS. In this case, after glutamate is added to glutamine tRNA, glutamine tRNA that has accepted glutamine can be generated by removing one amino group from glutamate.
13. Met to f-Met and Ser to Selenocysteine
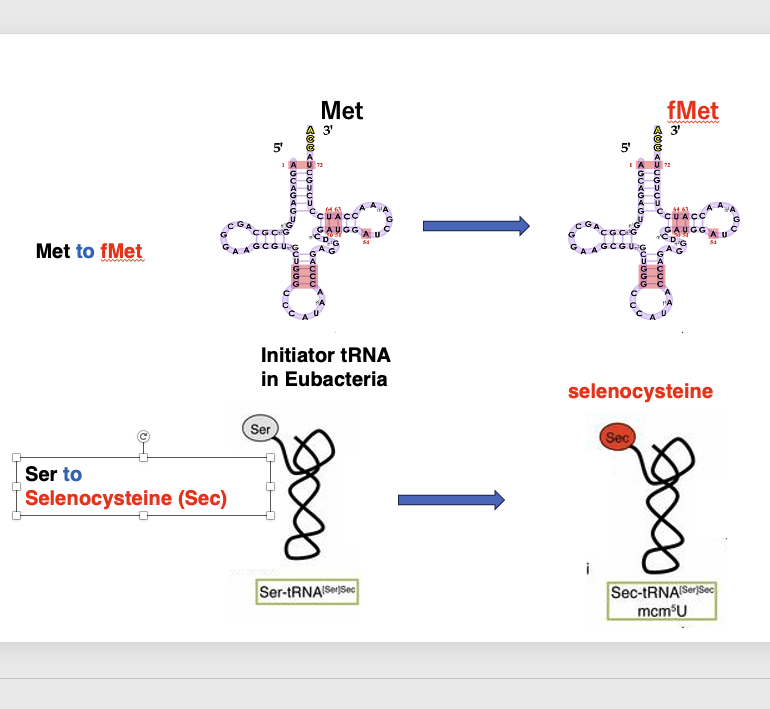
Let me discuss a more similar example. In the case of eubacterial initiator tRNA, methionine is first accepted, and then methionine is converted to formylmethionine on the tRNA. In the case of selenocysteine tRNA, serine is first added to selenocysteine tRNA. The added serine is converted to selenocysteine on the tRNA.
14. Pairing rule between Codon and Anticodon : Assign 64 codons to 20 amino acids
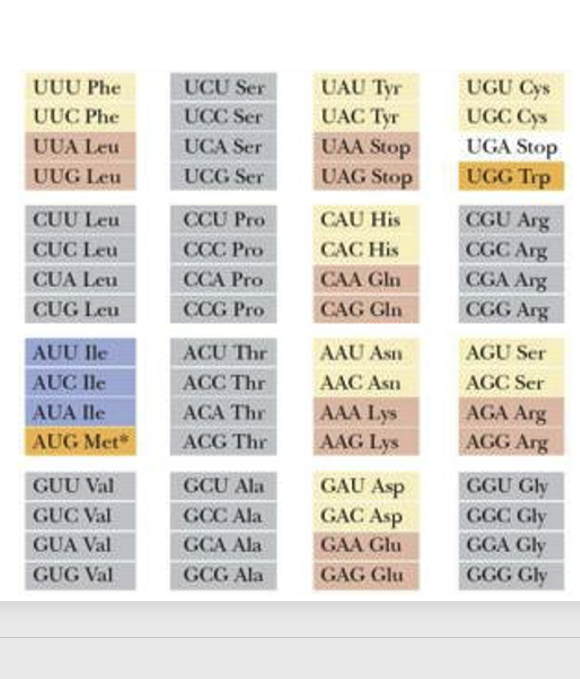
Regardless of whether they are bacteria, animals, plants, or viruses, all organisms on Earth use the same set of 20 amino acids. On the other hand, a system exists where a continuous sequence of three bases, called a codon, corresponds to one amino acid. Since there are 64 possible combinations of three consecutive bases, the correspondence between codons and the 20 amino acids is not one-to-one, meaning that different codons can specify the same amino acid.
This genetic code table is fundamentally applicable to both prokaryotes and eukaryotes. The reason for saying ‘fundamentally’ is that in the mitochondria of various species, the correspondence between codons and amino acids deviates from this genetic code table in some cases.
Furthermore, it is known that some prokaryotes and eukaryotes also exhibit deviations from this genetic code table.
15. Synonymous Codon, Family Box, Two codon set
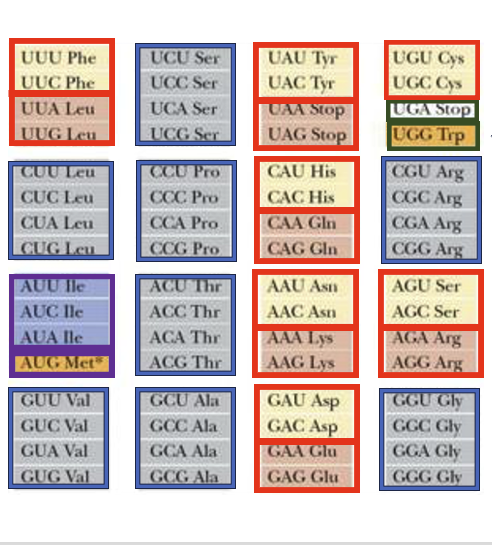
Here are the key points on the codon-anticodon pairing rules based on the genetic code table: The genetic code table shows which amino acid corresponds to each codon. For example, the codon UUU codes for the amino acid phenylalanine. This is because the transfer RNA carrying phenylalanine has an anticodon that can form stable hydrogen bonds with the UUU codon. A notable feature is the degeneracy of the genetic code, where multiple codons code for the same amino acid. This is seen in the “two-codon sets” where the third base can be U or C (or A or G) and still code for the same amino acid, e.g. phenylalanine, leucine, aspartic acid, and glutamic acid.
Another pattern is the “family boxes” , where any base at the third position codes for the same amino acid, e.g. the leucine codons starting with CU.
However, there are exceptions to these patterns. The isoleucine box has AUU, AUC, and AUA coding for isoleucine, but AUG coding for methionine. The cysteine codons UGU and UGC follow the two-codon set, but UGA codes for a stop signal and UGG codes for tryptophan, showing a special split.
In summary, while the genetic code exhibits patterns like two-codon sets and family boxes that allow some flexibility in codon-anticodon pairing, there are also exceptions that deviate from these general rules.
16. How to make stable bonds between codon and anticodon
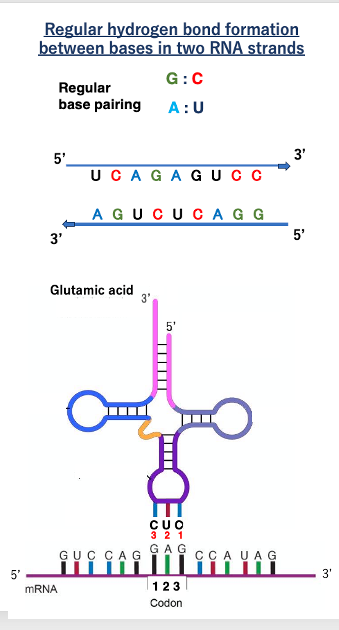
First, let us discuss the usual hydrogen bonding between RNA strands. Two RNA strands facing in opposite directions can form hydrogen bonds between bases: G can form a stable hydrogen bond with C, while A can form a stable hydrogen bond with U. This is the regular hydrogen bond formation rule.
This is an illustration of a transfer RNA charged with glutamic acid forming stable hydrogen bonds with codon GAG. Here is the messenger RNA, with the 5’-end on this side and the 3’ -end on the other side. For nucleic acids, the convention is to number the sequence from the 5’-end to the 3’-end. So, for GAG codon, the first base is G, the second is A, and the third is G.
The anticodon of the transfer RNA forming stable hydrogen bonds with this codon is CUC, with C as the first base, U as the second, and C as the third. For stable hydrogen bonding between bases in two nucleic acid molecules, the molecules must be antiparallel, if the messenger RNA is oriented in this direction, the tRNA must be oriented in the opposite direction.
17. Wobble rule : Special rule for base pairing of the third and first anticodons
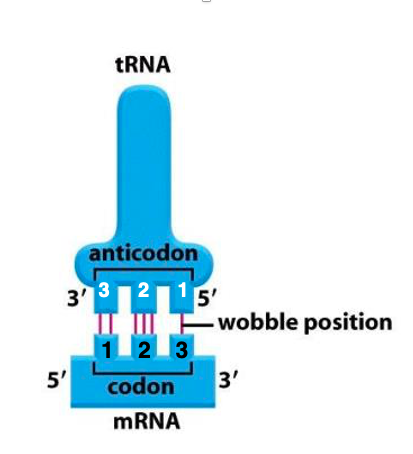
The usual base pairing between RNA bases is G and C, A and U. However, special rule applies to the base pairing of the third codon of messenger RNA, and the first anticodon of transfer RNA.
This is called the wobble rule. where wobble means fluctuation. Simply, the third codon and the first anticodon of transfer RNA are not mutually recognized so rigidly.
18. More about Wobble rule
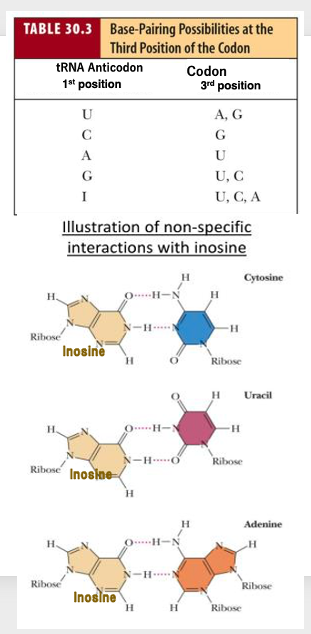
In Wobble rule, Uracil located at the 1st anticodon is allowed to bind to both A and G located at the third codon. In addition, when 1st anticodon is G, it can bind to U and C.
On the other hand, when the first anticodon is A, it can bind to only Uracil. if the first anticodon is C, only G can make stable pairing. It is also known that the first anticodon of transfer RNA contains various chemically modified bases.
For example, if the first base of trans-RNA is inosine, it can make hydrogen bonds with C, U, and A, as shown here.
19. Examples of the wobble rule : Two codon set
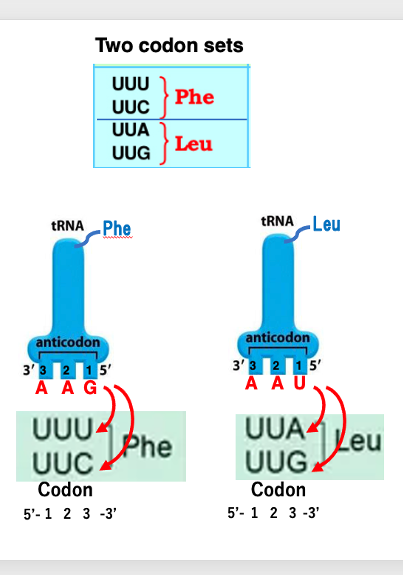
Let’s look at some more examples.
The transfer RNA with the anticodon GAA has phenylalanine attached to it. The first position of the anticodon and the third position of the codon follow the Wobble Rule, so the codons that can form stable hydrogen bonds with this anticodon are UUU or UUC.
Therefore, as long as the phenylalanine transfer RNA with the anticodon GAA exists in the cell, both the UUU and UUC codons correspond to phenylalanine.
The transfer RNA with the anticodon UAA has leucine attached to it.So, the codons that can form stable hydrogen bonds with this anticodon are UUA and UUG.
Since the leucine transfer RNA can stably bind to the UUA and UUG codon, both UUA and UUG are leucine codons.
20. Examples of the wobble rule : Family box
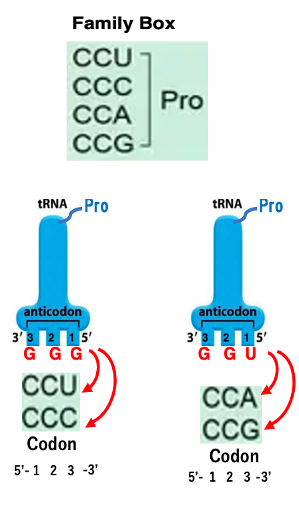
The transfer RNA with the anticodon GGG has proline attached to it. This transfer RNA can form stable hydrogen bonds with the codons CCU and CCC due to the Wobble Rule, which allows non-canonical base pairing between the first position of the anticodon and the third position of the codon.
Similarly, the transfer RNA with the anticodon UGG also has proline attached. This anticodon can stably hydrogen bond with the codons CCA and CCG.
Therefore, regardless of the third position of the codon, if the first two positions are CC, either of the two proline transfer RNAs (with anticodons GGG or UGG), will correspond to it. This means that the codon family CCN (where N can be any base) specifies for proline.
21. Anticodon bases of tRNAs for Ile, Met, and Trp
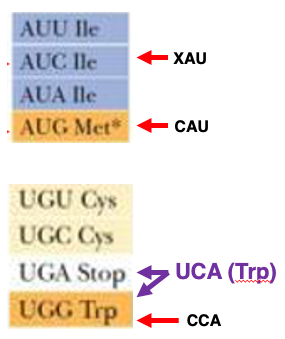
This is the box that specifies isoleucine. The codons AUU, AUC, and AUA are codons for isoleucine. It is known that there exists an isoleucine transfer RNA that can form stable hydrogen bonds with any of these three codons. The first position of the anticodon of this isoleucine transfer RNA undergoes a very special base modification. For the methionine codon AUG, the anticodon of the corresponding transfer RNA is CAU, so it can only correspond to the AUG codon.
For the tryptophan codon UGG, the anticodon of the corresponding transfer RNA is CCA. AAA, Since the anticodon is CCA, it cannot correspond to the UGA codon, meaning only the UGG codon corresponds to tryptophan. If a tryptophan transfer RNA with the anticodon UCA were to appear in the cell, the first position being U would allow it to pair with both A and G. This means not only UGG but also UGA would become codons for tryptophan.
22. How the termination codons UAA, UAG, and UG terminate translation
Next topic is how the termination codons UAA, UAG, and UGA terminate translation.
There are no transfer RNAs having an anticodon that can stably pair with a termination codon. A protein, Release Factor binds to the termination codon and releases the nascent peptide from the transfer RNA.
23. Outline of translation steps to understand Stop codons
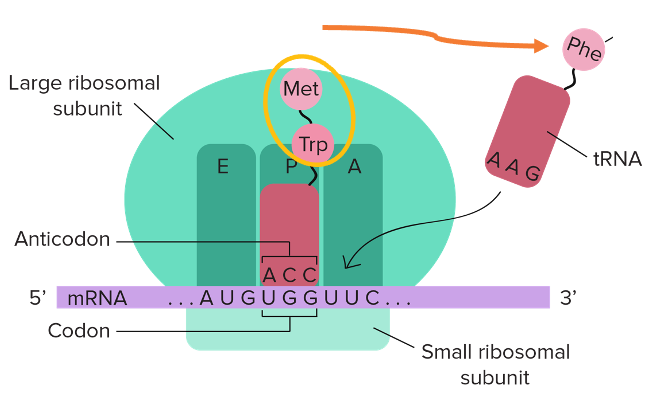
First, a brief description of the general steps of translation. Ribosome has three spaces, and each of it can accommodate one transfer RNA. They are named E-site, P-site, and A-site, in that order.
The transfer RNA in the P-site has a growing nascent peptide attached to it. A new transfer RNA that has accepted an amino acid is added to the A site. When a transfer RNA with the anticodon corresponding to the A site codon is inserted, the transfer RNA in the P site transfers its nascent peptide to the transfer RNA in the A site. This reaction increases the amino acid residues in the newborn peptide by one.
In this figure, the number of amino acid residues will be increased to 3 peptides by the addition of phenylalanine to make a tripeptide consisting of methionine-Tryptophane-Phenylalanine.
24. Termination reaction of translation
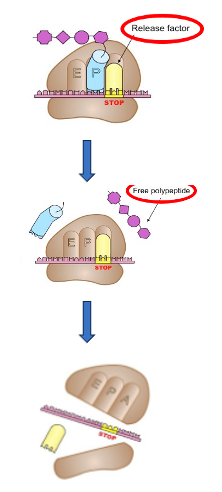
Termination reaction occurs, when ribosomal A site reaches a stop codon. No transfer RNA exists in the cell that can form a stable bond with the stop codon in the ribosome.
On the other hand, release factor recognizes the Stop codon and forms a stable bond with a termination codon. Then, hydrolysis of the ester bond between the ribose and amino acid of the peptidyl-tRNA is induced, and the nascent peptide is dissociated from the tRNA.
At the same time, the complex of the ribosome, mRNA, and release factor is disassembled using the energy of GTP. This is the termination of translation reaction.
25. Three-dimensional structure of the release factor
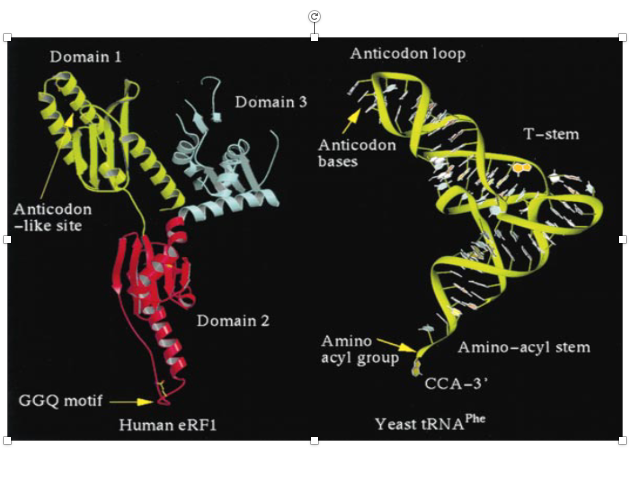
The three-dimensional structure of the release factor is known to be similar to that of transfer RNA. This is a general 3D model of transfer RNA, but if you look at the 3D structure of the release factor made of protein, you can see that the 3D structure of the two molecules is very similar, although the molecular elements that make up the 3D structure are different.
26. Initiator and Elongator tRNAs
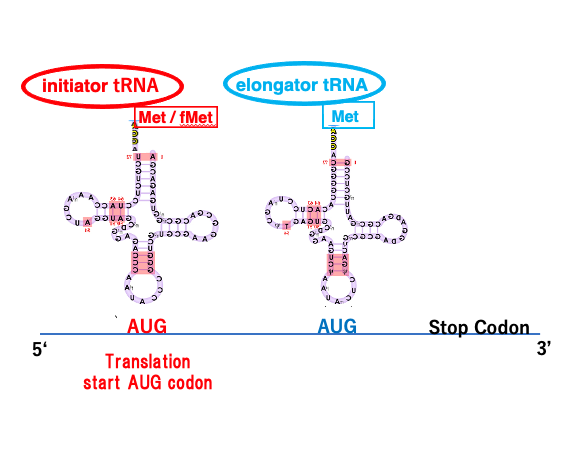
The codon for translation initiation is AUG, and the transfer RNA that corresponds to this codon is called an initiator transfer RNA on which methionine or formyl-methionine is charged, while transfer RNA that corresponds to non-translation initiation AUG codons are called elongator transfer RNA, that always charging methionine.
27. Initiator tRNA in Eukaryotes and Archaea
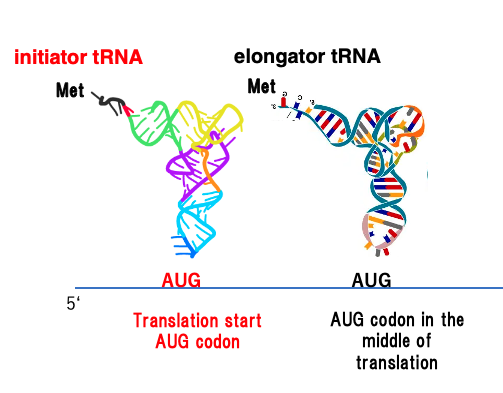
This figure schematically represents the translation initiation process in eukaryotes and archaea.
The amino acid carried by the initiator transfer RNA is methionine. Elongator transfer RNA also carries methionine. Therefore, in eukaryotes and archaea, the first amino acid of a polypeptide is methionine, and the amino acid corresponding to the internal AUG codons is also methionine.
28. Initator tRNA in Eubacteria, Chloroplasts, and Mitochondria
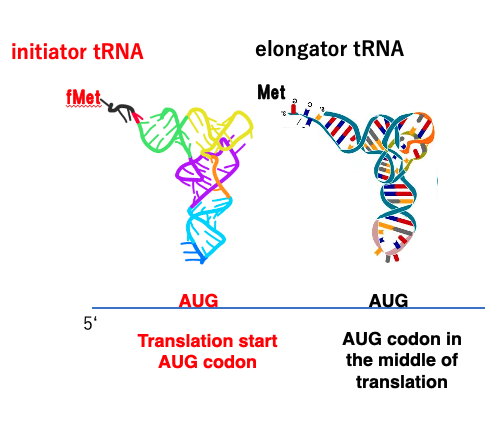
On the other hand, the initiation of translation in eubacteria and chloroplast, mitochondria is slightly different.
In these organisms, the initiator tRNA has formylmethionine instead of methionine. On the other hand, the elongator tRNA is charging methionine.
29. Amino acid conversion on Initiator tRNA in Eubacteria: Met to f-Met
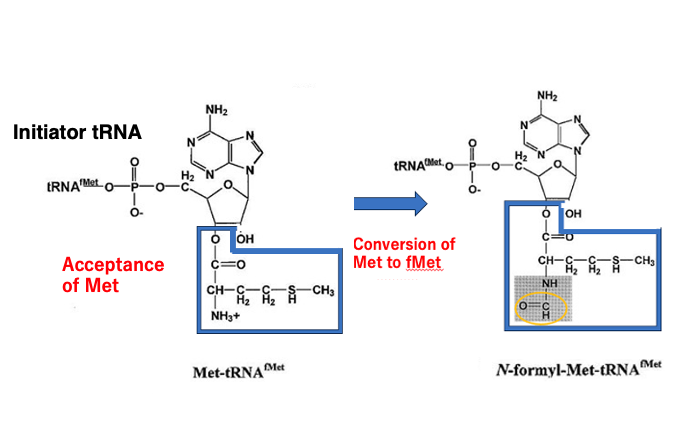
I will explain on the formylmethionine acceptance of the initiator transfer RNA in eubacteria. It is very interesting that methionine is first added to this initiator transfer RNA, but it is not formylmethionine. This means that amino acid modification occurs on the initiator transfer RNA accepting methionine to charge formylmethionine.
On the initiator transfer RNA, methionine undergoes formylation and is converted to formylmethionine. I have already explained the similar phenomena. In some eubacteria, glutamine-receptor transfer RNA and asparagine-receptor transfer RNA first accept aspartic acid, or glutamic acid, which is then converted to glutamine or asparagine on transfer RNA.
コメント
コメント一覧 (1件)
Hi, this is a comment.
To get started with moderating, editing, and deleting comments, please visit the Comments screen in the dashboard.
Commenter avatars come from Gravatar.